-
oa Mixed Platinum Metal Oxide Catalysts
Activity Patterns in Alloy Systems
- Source: Platinum Metals Review, Volume 10, Issue 1, Jan 1966, p. 10 - 13
-
- 01 Jan 1966
Preview this article:
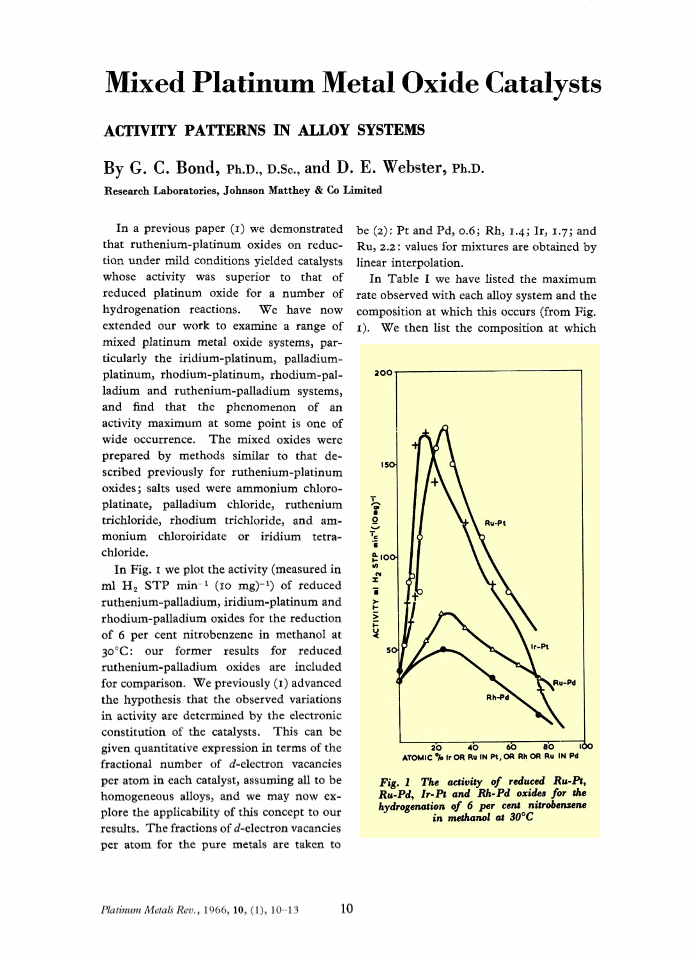



Mixed Platinum Metal Oxide Catalysts, Page 1 of 1
< Previous page Next page > /docserver/preview/fulltext/pmr/10/1/pmr0010-0010-1.gif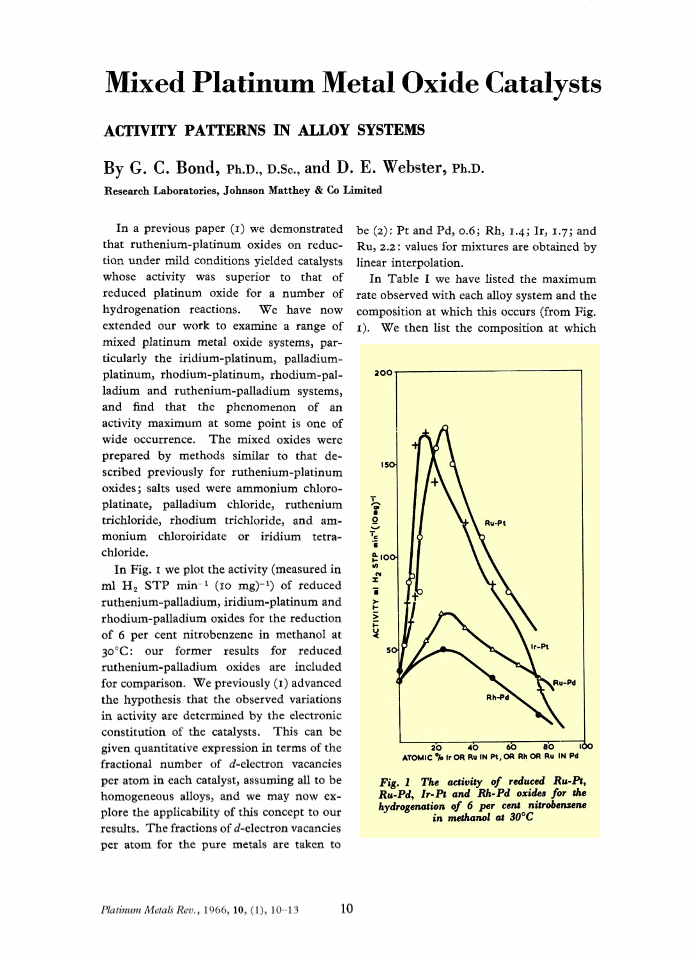
There is no abstract available.
© Johnson Matthey