-
oa High Temperature Behaviour of Iridium-Rhodium Alloys
Oxidation Resistance and Compatibility
- Source: Platinum Metals Review, Volume 11, Issue 2, Apr 1967, p. 53 - 55
-
- 01 Jan 1967
Preview this article:
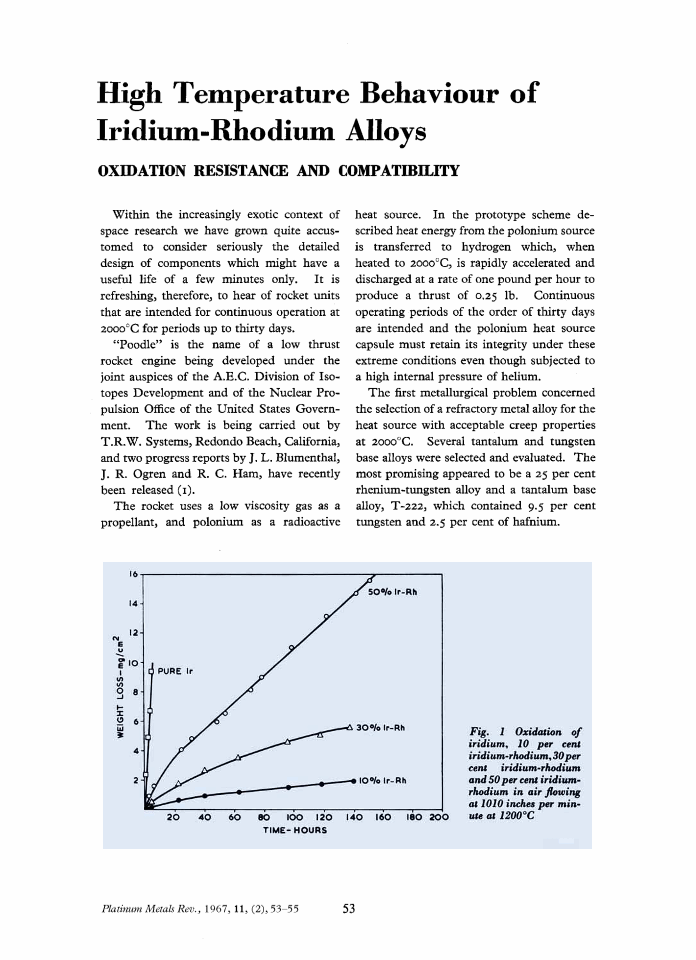



High Temperature Behaviour of Iridium-Rhodium Alloys, Page 1 of 1
< Previous page Next page > /docserver/preview/fulltext/pmr/11/2/pmr0011-0053-1.gif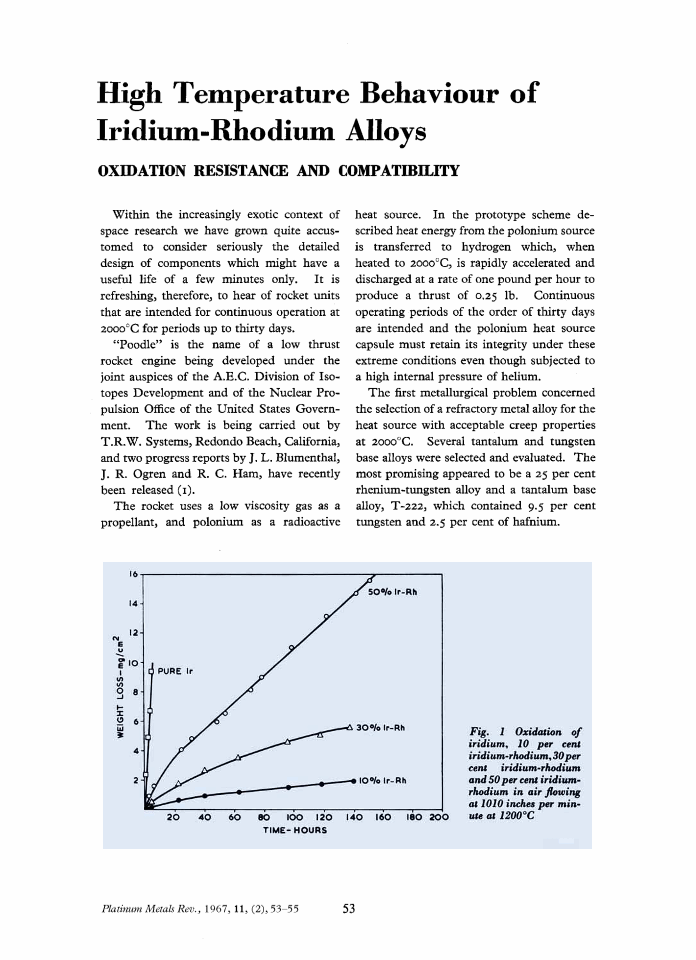
There is no abstract available.
© Johnson Matthey