-
oa Platinum Catalysts for the Control of Air Pollution
A Tail Gas Reduction System for Nitric Acid Plants
- Source: Platinum Metals Review, Volume 12, Issue 1, Jan 1968, p. 2 - 6
-
- 01 Jan 1968
Preview this article:
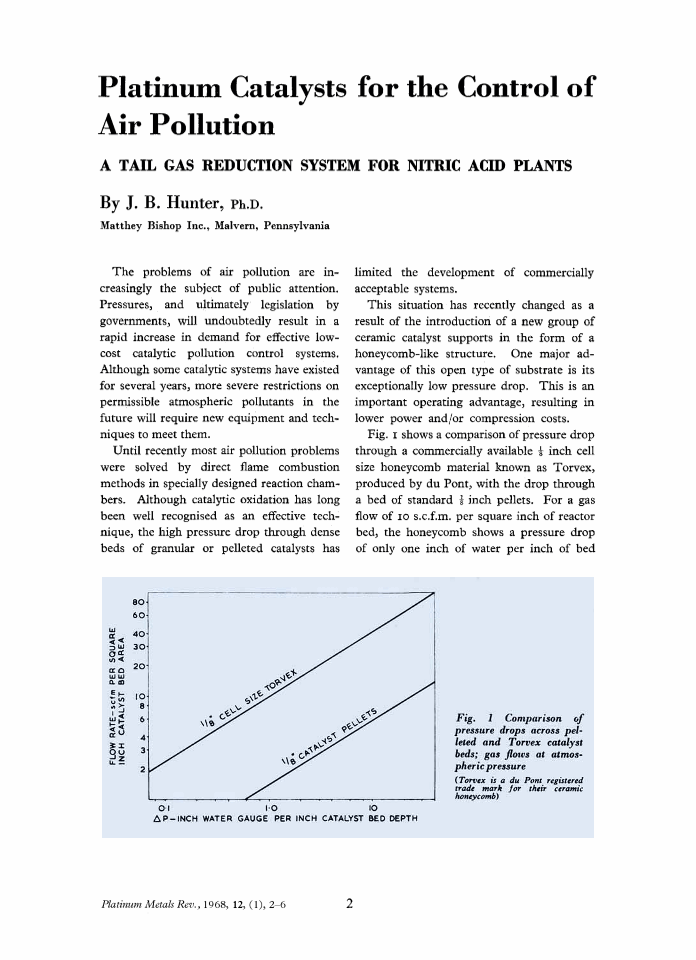



Platinum Catalysts for the Control of Air Pollution, Page 1 of 1
< Previous page Next page > /docserver/preview/fulltext/pmr/12/1/pmr0012-0002-1.gif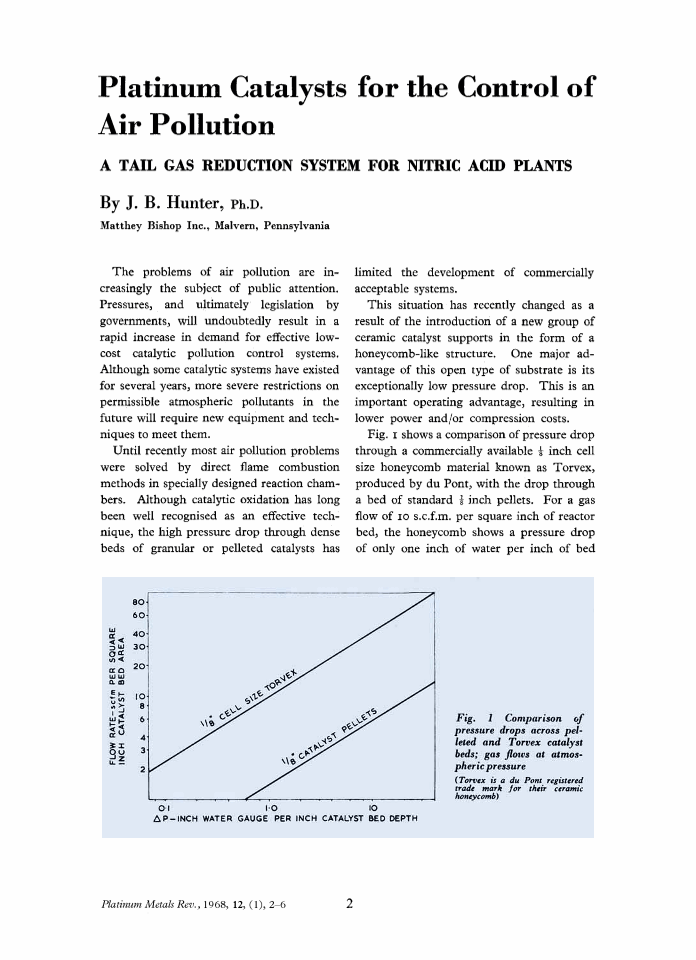
There is no abstract available.
© Johnson Matthey