-
oa Tertiary Phosphine Complexes of the Platinum Metals
Principles of their Reaction Mechanisms and Uses in Homogeneous Catalysis
- Source: Platinum Metals Review, Volume 12, Issue 2, Apr 1968, p. 50 - 53
-
- 01 Jan 1968
Preview this article:
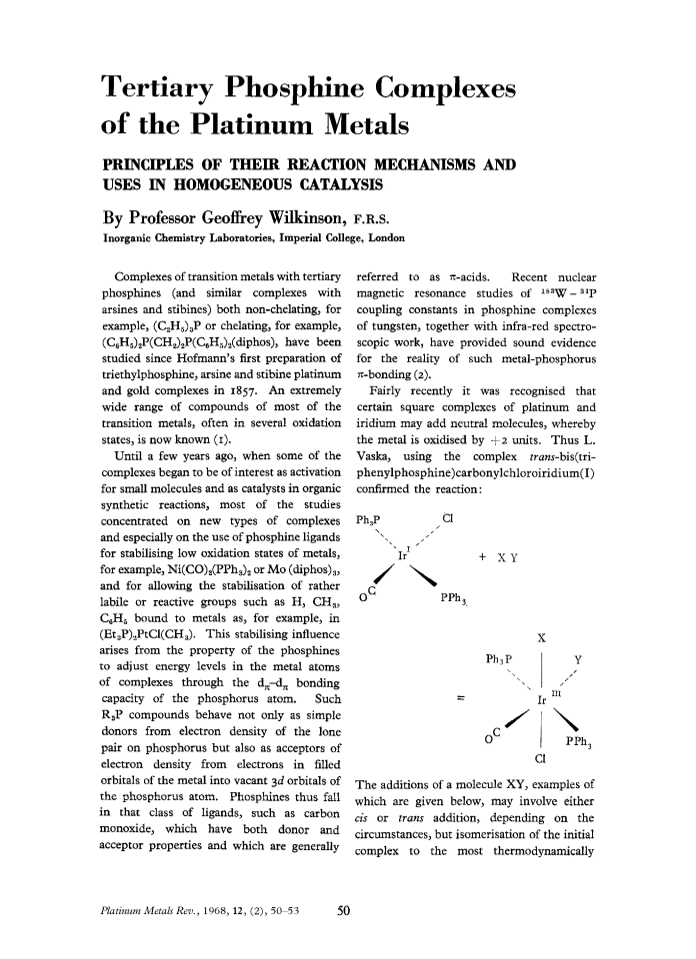



Tertiary Phosphine Complexes of the Platinum Metals, Page 1 of 1
< Previous page Next page > /docserver/preview/fulltext/pmr/12/2/pmr0012-0050-1.gif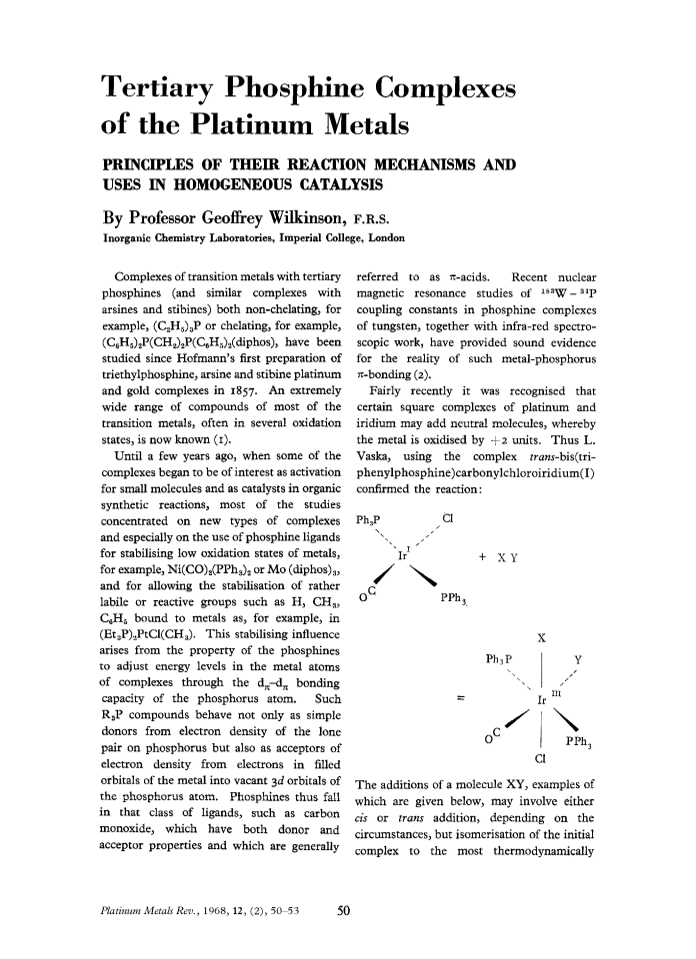
There is no abstract available.
© Johnson Matthey