-
oa Palladium Alloy Diffusion Units
A New Range of Commercial Equipment for the Production of Ultra-pure Hydrogen
- Source: Platinum Metals Review, Volume 13, Issue 4, Oct 1969, p. 141 - 145
-
- 01 Jan 1969
Preview this article:
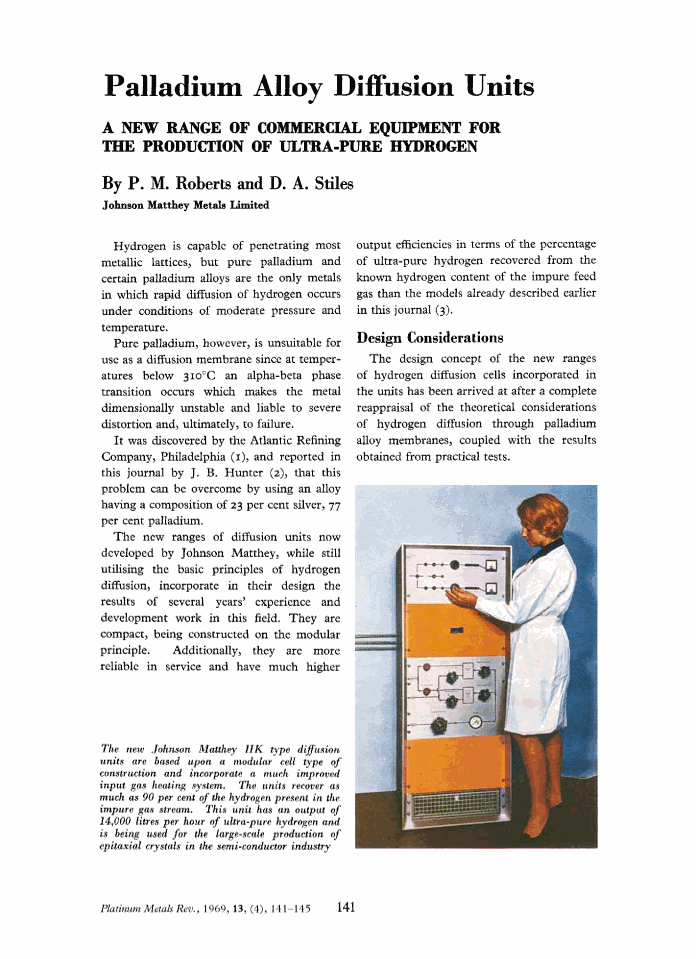



Palladium Alloy Diffusion Units, Page 1 of 1
< Previous page Next page > /docserver/preview/fulltext/pmr/13/4/pmr0013-0141-1.gif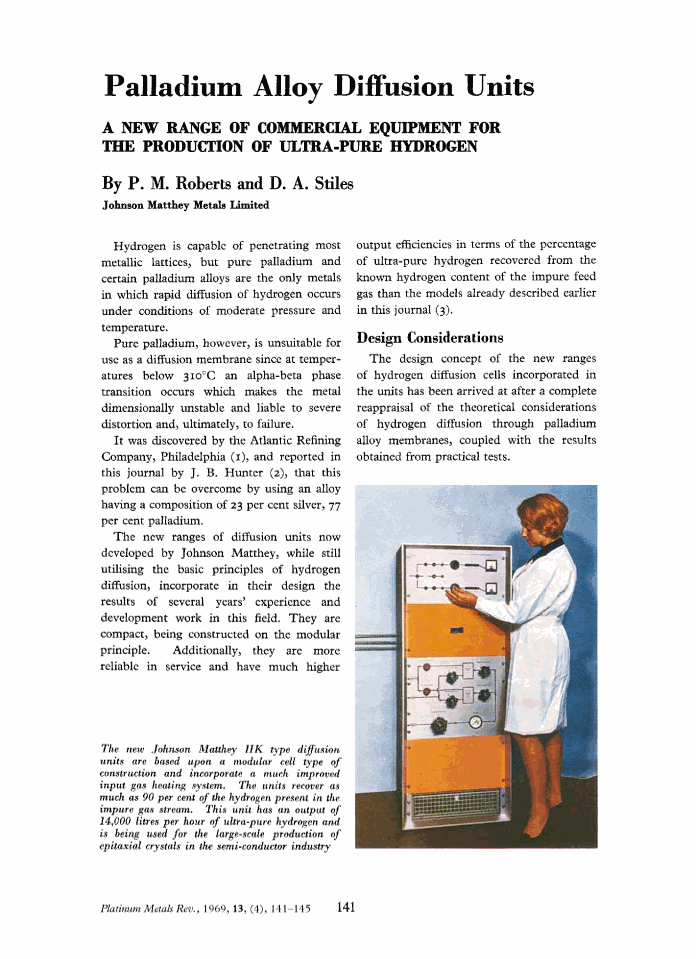
There is no abstract available.
© Johnson Matthey