-
oa Complexes of Pyridine Bases with Rhodium
Redox Behaviour and Antibacterial Activity
- Source: Platinum Metals Review, Volume 14, Issue 2, Apr 1970, p. 50 - 53
-
- 01 Jan 1970
Preview this article:
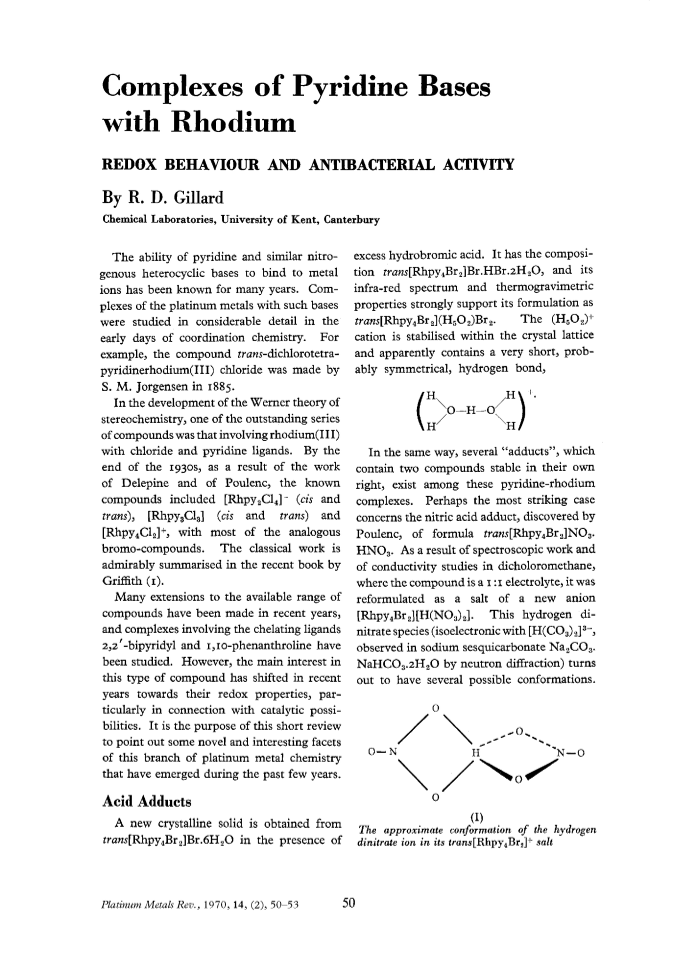



Complexes of Pyridine Bases with Rhodium, Page 1 of 1
< Previous page Next page > /docserver/preview/fulltext/pmr/14/2/pmr0014-0050-1.gif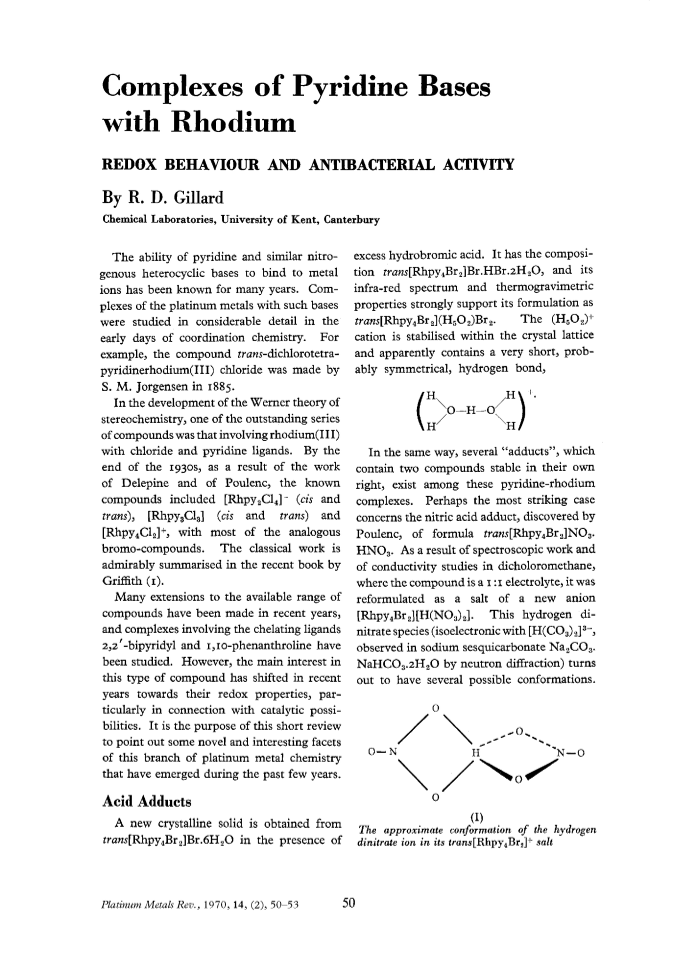
There is no abstract available.
© Johnson Matthey