-
oa Directional Electrical Conductivity in Platinum Complexes
Models for Room Temperature Superconductivity
- Source: Platinum Metals Review, Volume 15, Issue 4, Oct 1971, p. 129 - 131
-
- 01 Jan 1971
Preview this article:
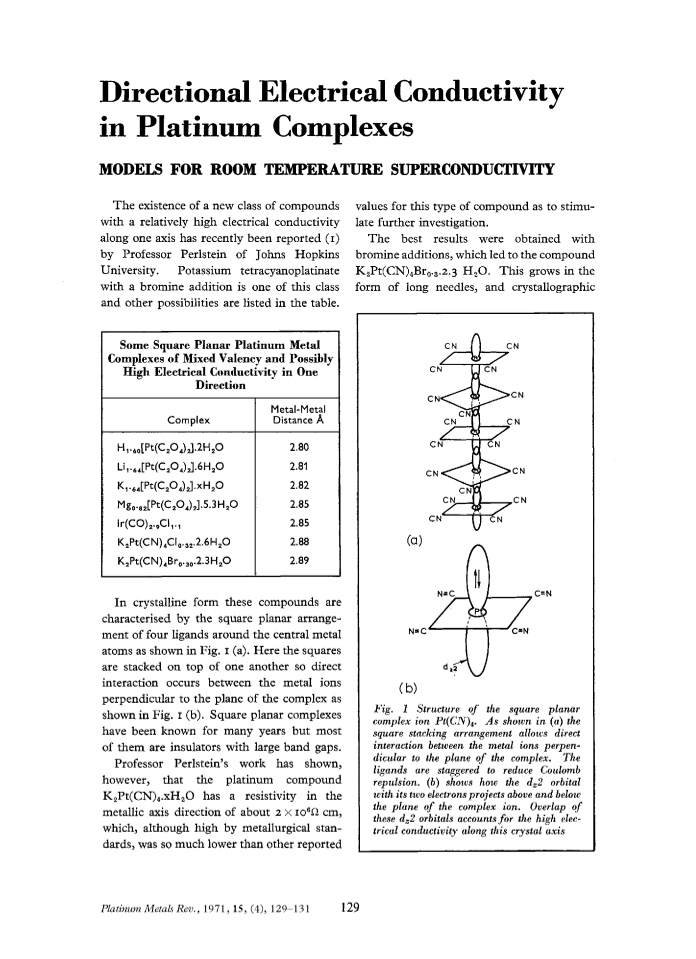



Directional Electrical Conductivity in Platinum Complexes, Page 1 of 1
< Previous page Next page > /docserver/preview/fulltext/pmr/15/4/pmr0015-0129-1.gif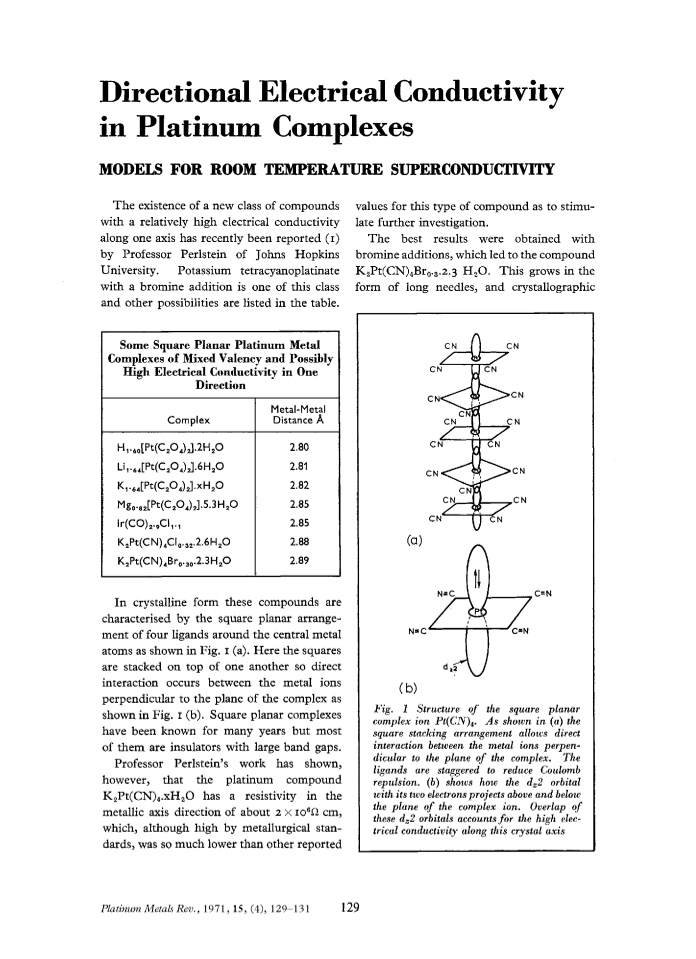
There is no abstract available.
© Johnson Matthey