-
oa Vitreous Palladium Alloys
Low-Temperature Measurement in a Nuclear Environment
- Source: Platinum Metals Review, Volume 16, Issue 3, Jul 1972, p. 91 - 93
-
- 01 Jan 1972
Preview this article:
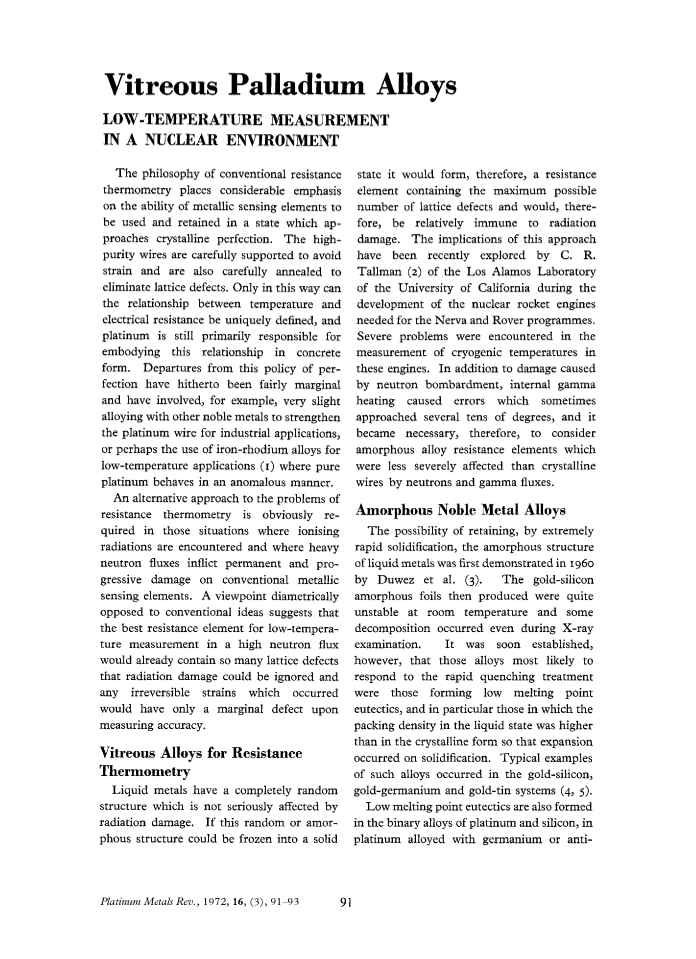



Vitreous Palladium Alloys, Page 1 of 1
< Previous page Next page > /docserver/preview/fulltext/pmr/16/3/pmr0016-0091-1.gif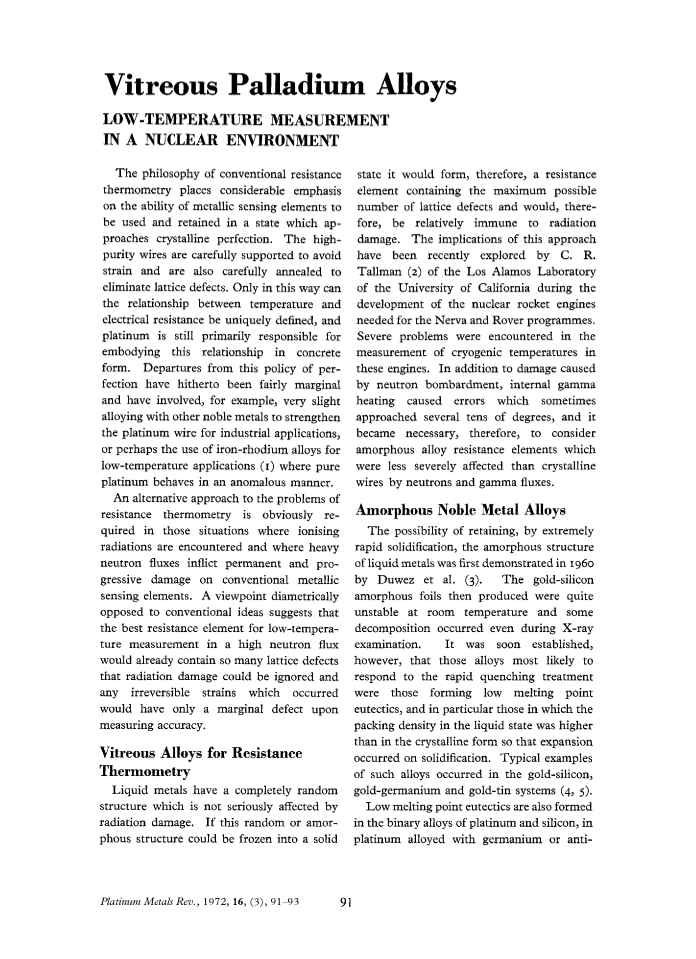
There is no abstract available.
© Johnson Matthey