-
oa Coordination Compounds of the Platinum Group Metals
A Review of their Preparative Methods and Applications
- Source: Platinum Metals Review, Volume 18, Issue 4, Oct 1974, p. 122 - 129
-
- 01 Jan 1974
- Previous Article
- Table of Contents
- Next Article
Preview this article:
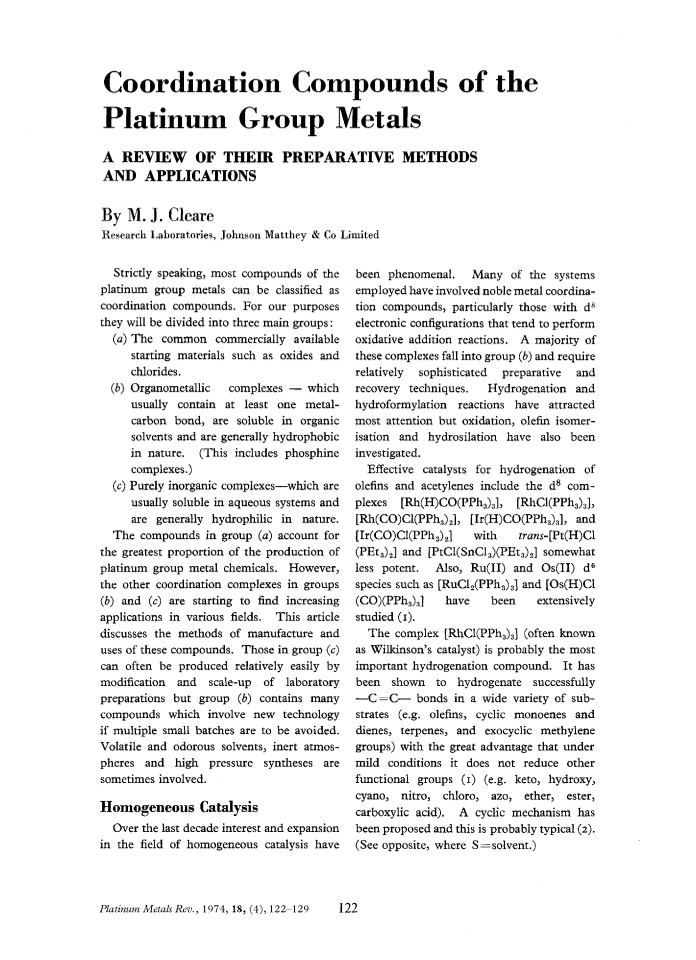



Coordination Compounds of the Platinum Group Metals, Page 1 of 1
< Previous page Next page > /docserver/preview/fulltext/pmr/18/4/pmr0018-0122-1.gif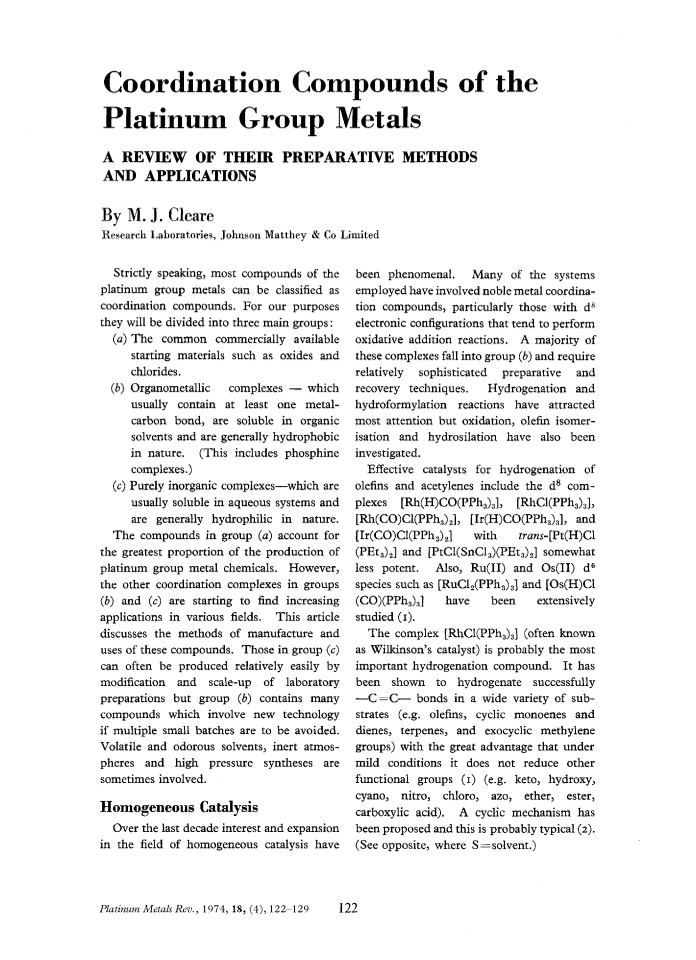
There is no abstract available.
© Johnson Matthey