-
oa The Diffusion of Hydrogen through Palladium
- Source: Platinum Metals Review, Volume 2, Issue 1, Jan 1958, p. 16 - 22
-
- 01 Jan 1958
Preview this article:
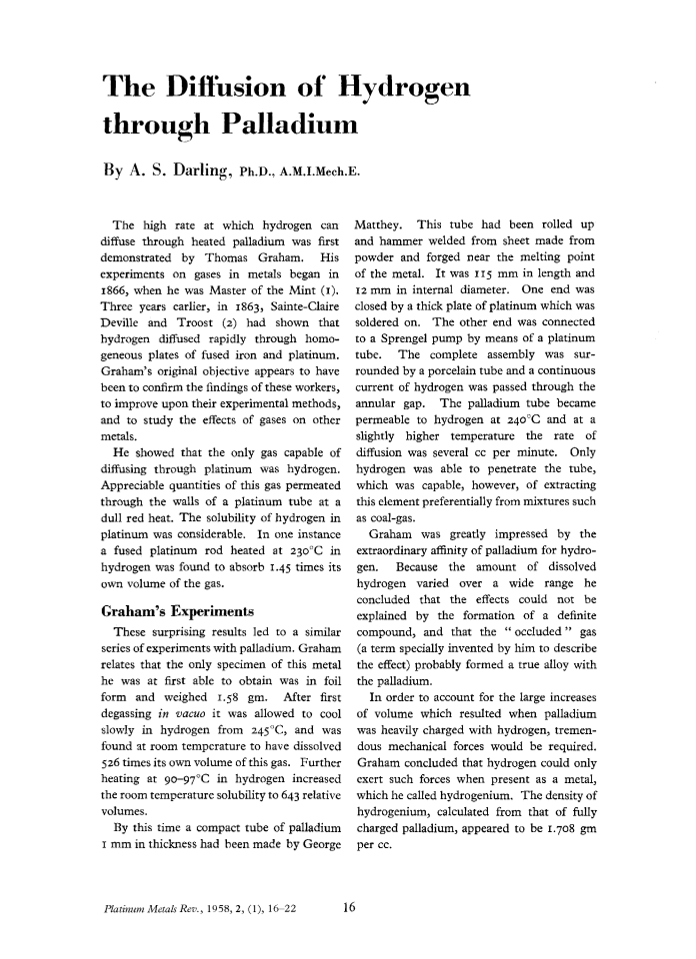



The Diffusion of Hydrogen through Palladium, Page 1 of 1
< Previous page Next page > /docserver/preview/fulltext/pmr/2/1/pmr0002-0016-1.gif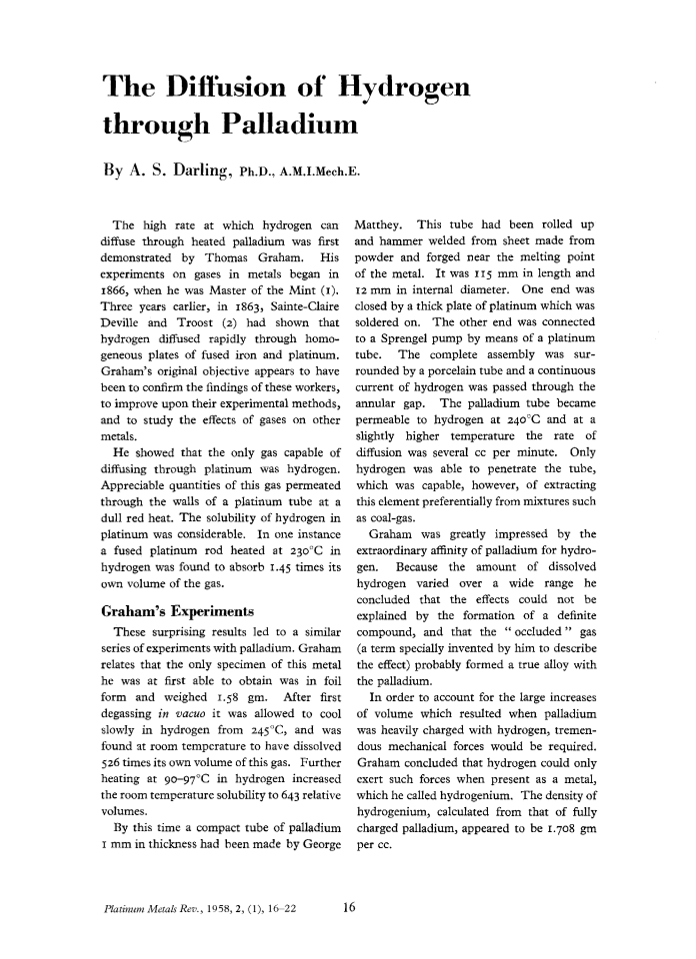
There is no abstract available.
© Johnson Matthey