-
oa Platinum and Palladium in the Pharmaceutical Industry
- Source: Platinum Metals Review, Volume 2, Issue 3, Jul 1958, p. 86 - 89
-
- 01 Jan 1958
Preview this article:
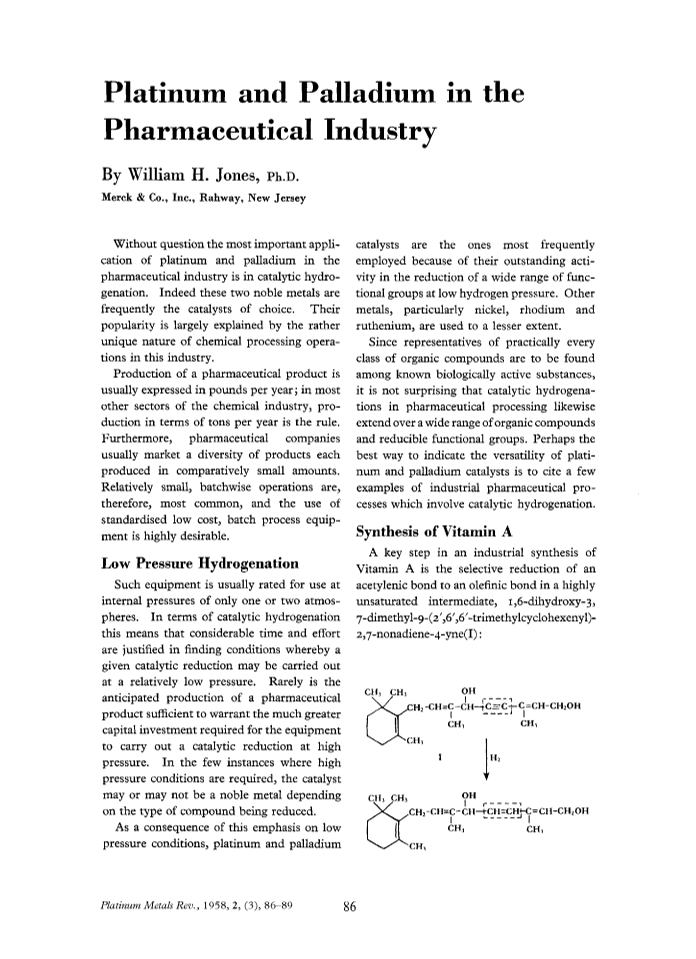



Platinum and Palladium in the Pharmaceutical Industry, Page 1 of 1
< Previous page Next page > /docserver/preview/fulltext/pmr/2/3/pmr0002-0086-1.gif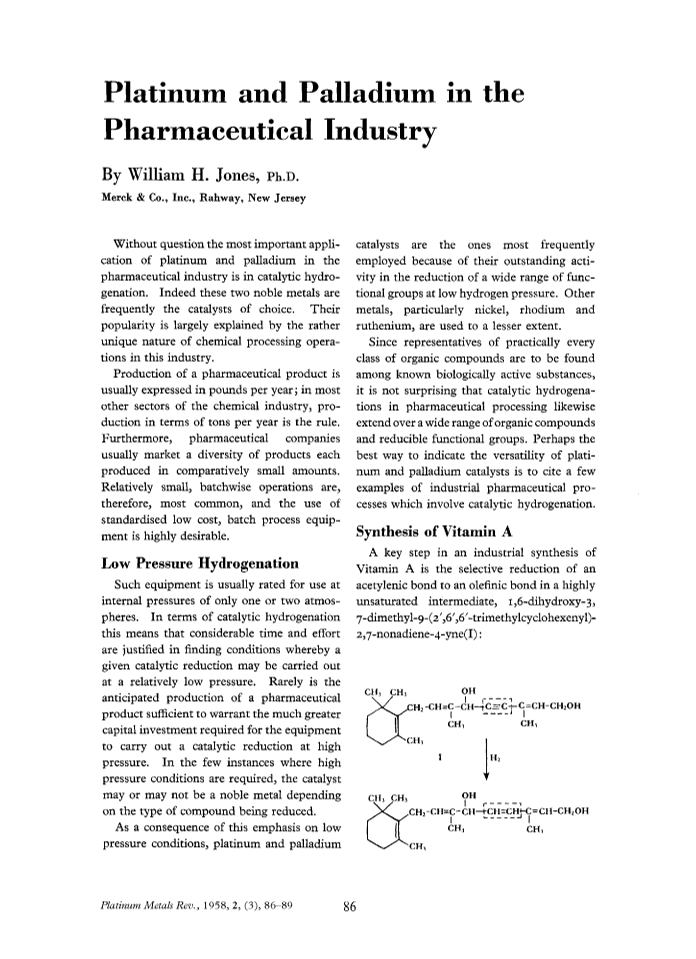
There is no abstract available.
© Johnson Matthey