-
oa Choosing Catalysts for Selective Behaviour
A Graphical Method of Studying Reactions
- Source: Platinum Metals Review, Volume 2, Issue 4, Oct 1958, p. 124 - 127
-
- 01 Jan 1958
Preview this article:
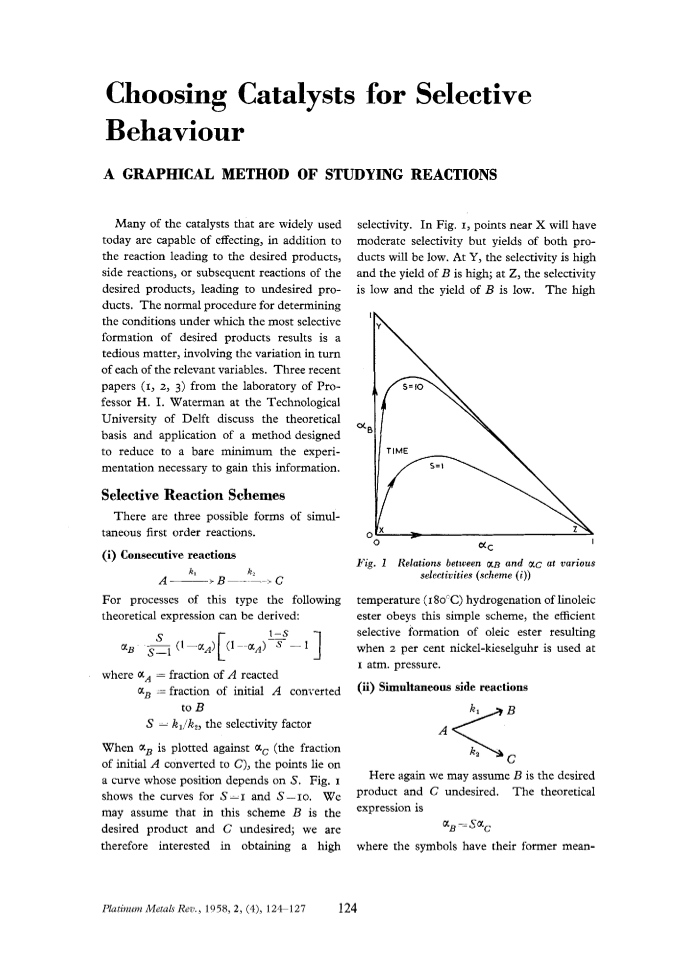



Choosing Catalysts for Selective Behaviour, Page 1 of 1
< Previous page Next page > /docserver/preview/fulltext/pmr/2/4/pmr0002-0124-1.gif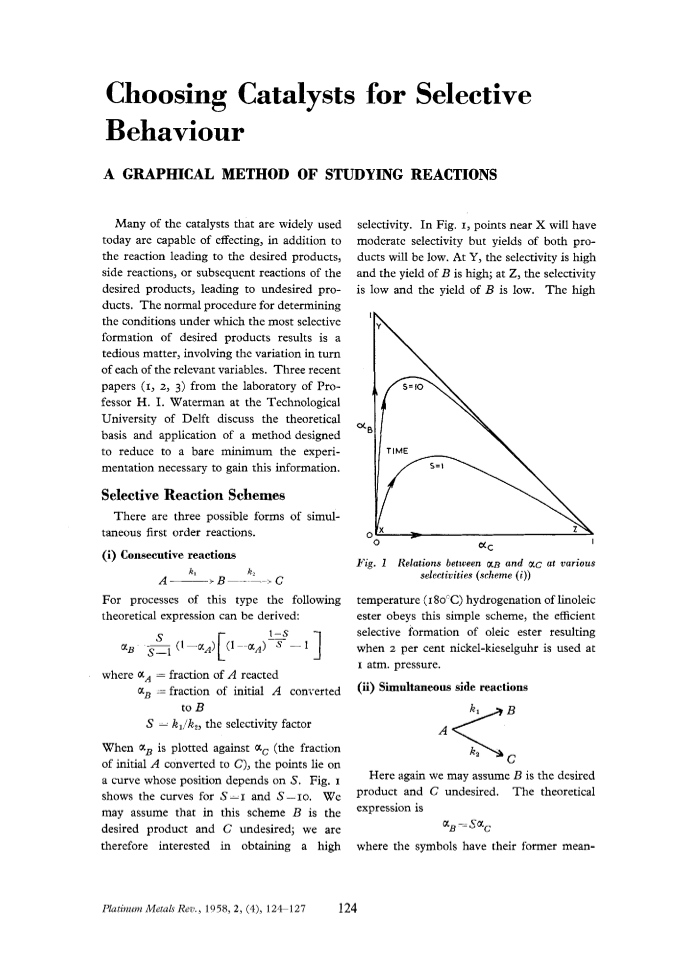
There is no abstract available.
© Johnson Matthey