-
oa Iridium Based Ultramicroelectrodes
Development and use in Electrochemical Analysis
- Source: Platinum Metals Review, Volume 34, Issue 3, Jul 1990, p. 131 - 134
-
- 01 Jul 1990
Preview this article:
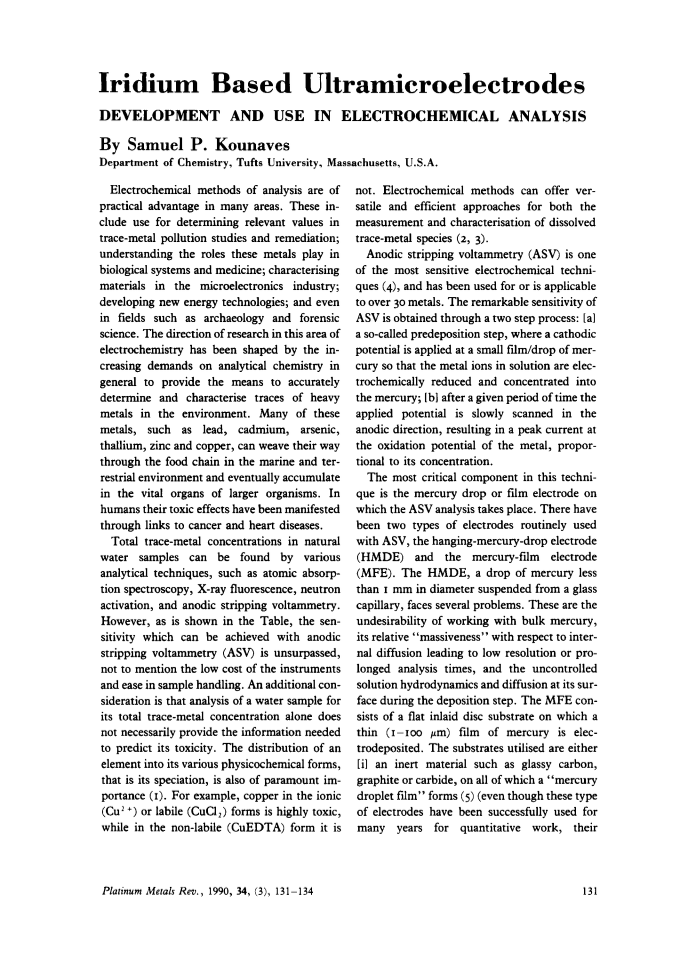



Iridium Based Ultramicroelectrodes, Page 1 of 1
< Previous page Next page > /docserver/preview/fulltext/pmr/34/3/pmr0034-0131-1.gif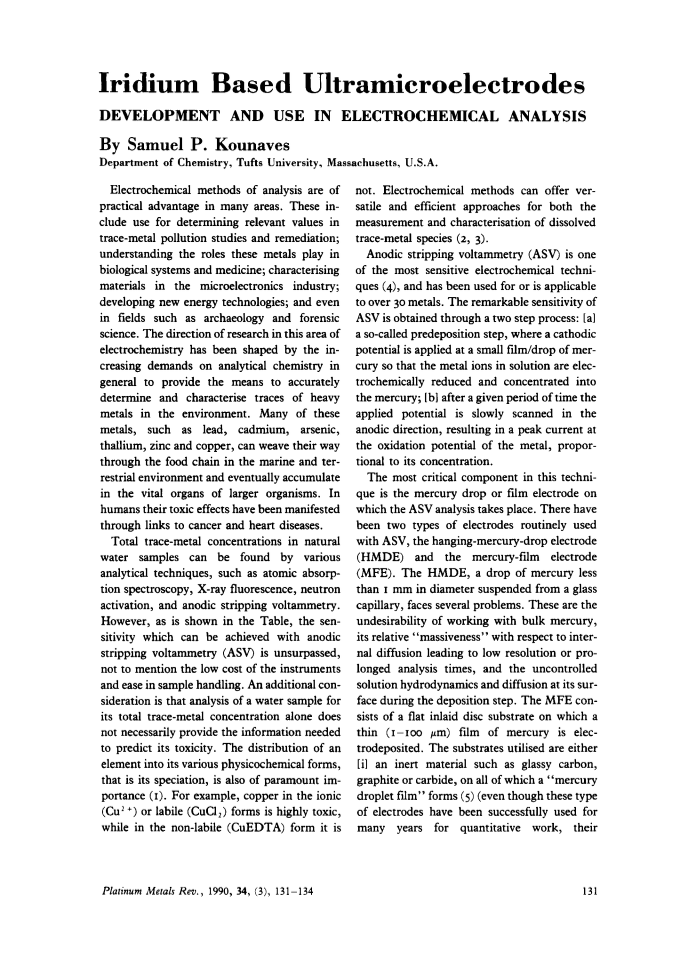
There is no abstract available.
© Johnson Matthey