-
oa Palladium Supported Catalysts in CO + RONO Reactions
- Source: Platinum Metals Review, Volume 34, Issue 4, Oct 1990, p. 178 - 180
-
- 01 Oct 1990
Preview this article:
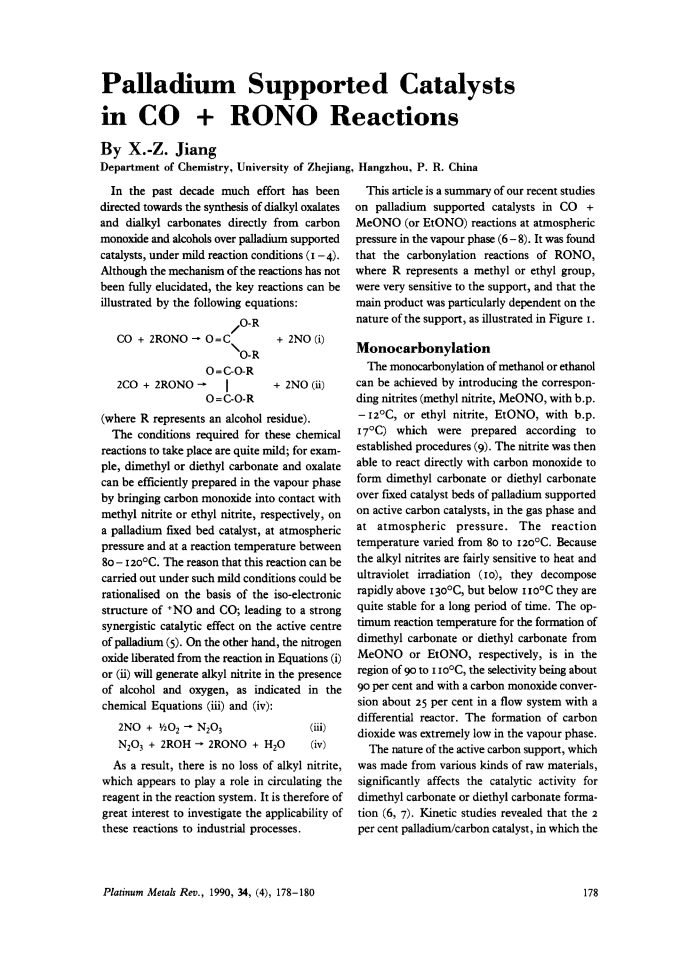



Palladium Supported Catalysts in CO + RONO Reactions, Page 1 of 1
< Previous page Next page > /docserver/preview/fulltext/pmr/34/4/pmr0034-0178-1.gif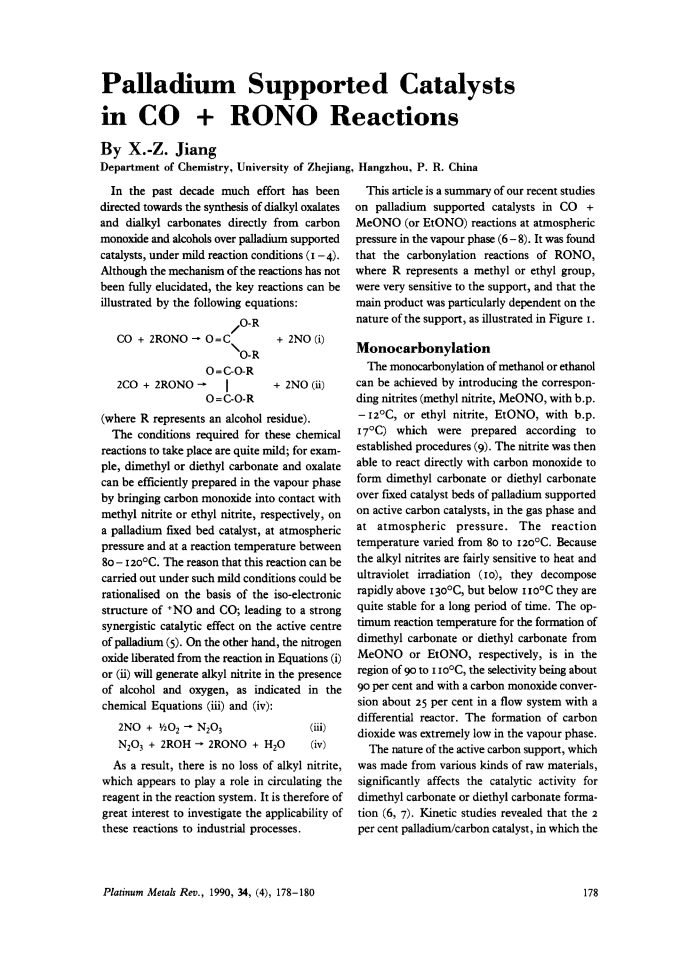
There is no abstract available.
© Johnson Matthey