-
oa Solvated Atoms of Platinum, Palladium and Gold
Precursors to Colloids, Films and Catalysts
- Source: Platinum Metals Review, Volume 36, Issue 2, Apr 1992, p. 80 - 84
-
- 01 Jan 1992
Preview this article:
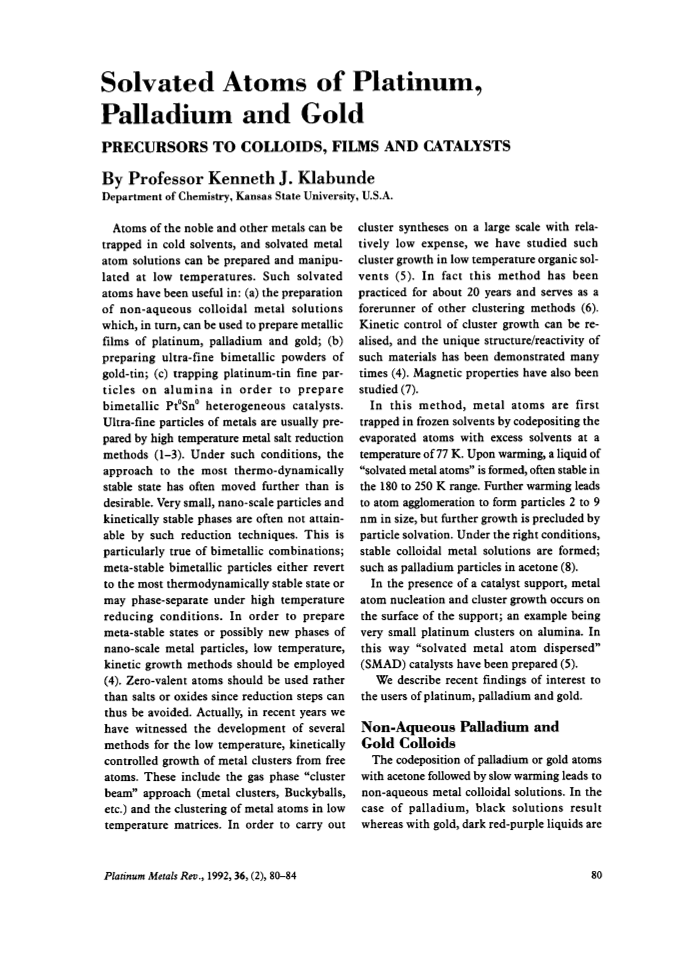



Solvated Atoms of Platinum, Palladium and Gold, Page 1 of 1
< Previous page Next page > /docserver/preview/fulltext/pmr/36/2/pmr0036-0080-1.gif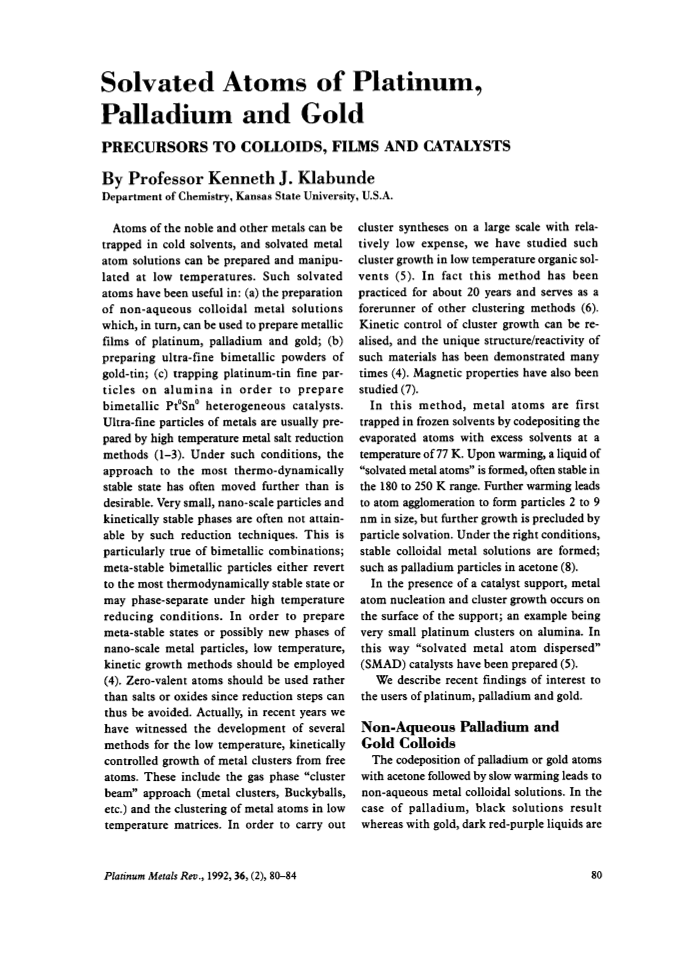
There is no abstract available.
© Johnson Matthey