-
oa Supported Palladium Catalysts
A Comparison of Solvated Metal Atom Impregnated and Conventionally Prepared Catalysts
- Source: Platinum Metals Review, Volume 37, Issue 2, Apr 1993, p. 72 - 75
-
- 01 Jan 1993
Preview this article:
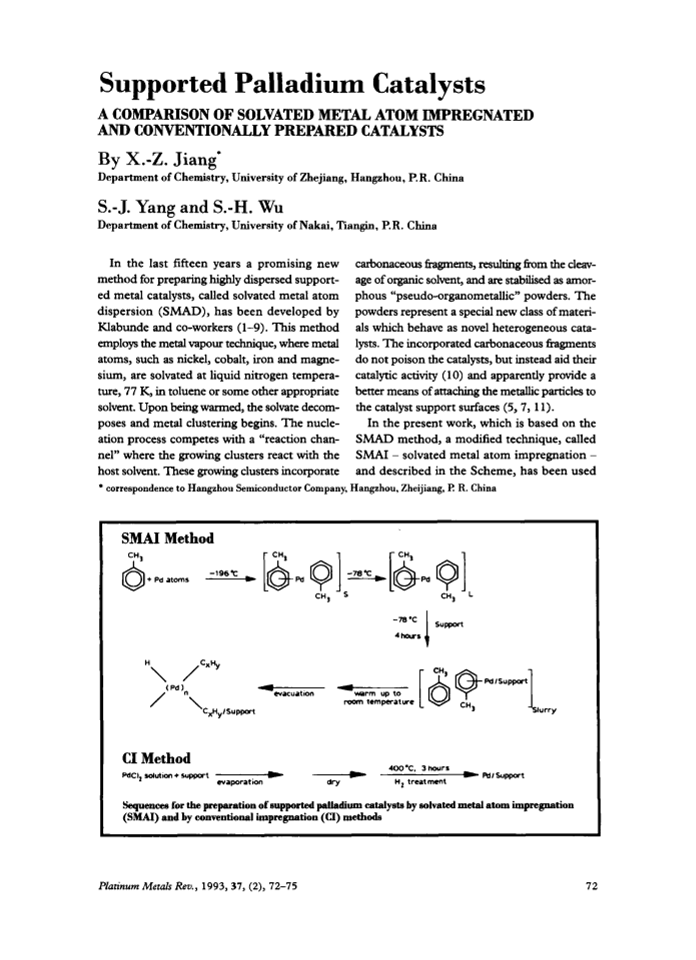



Supported Palladium Catalysts, Page 1 of 1
< Previous page Next page > /docserver/preview/fulltext/pmr/37/2/pmr0037-0072-1.gif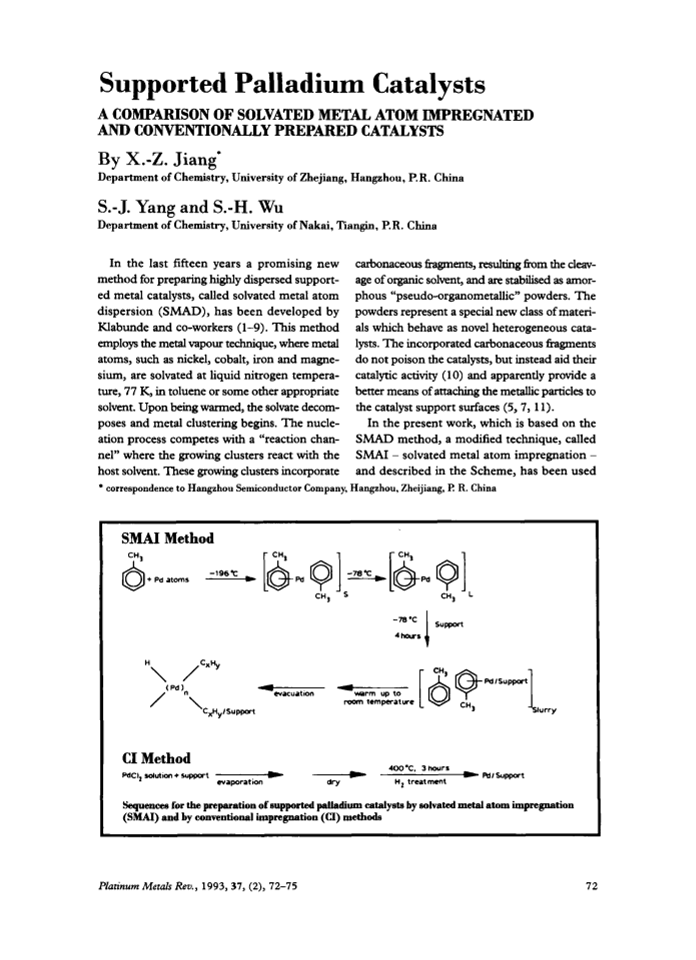
There is no abstract available.
© Johnson Matthey