-
oa Hydrogen Interstitial Structures in Palladium-Silver Membranes
Elastic Energy Dissipation Indications of Deviations from Expected Homogeneity
- Source: Platinum Metals Review, Volume 37, Issue 4, Oct 1993, p. 220 - 222
-
- 01 Jan 1993
Preview this article:
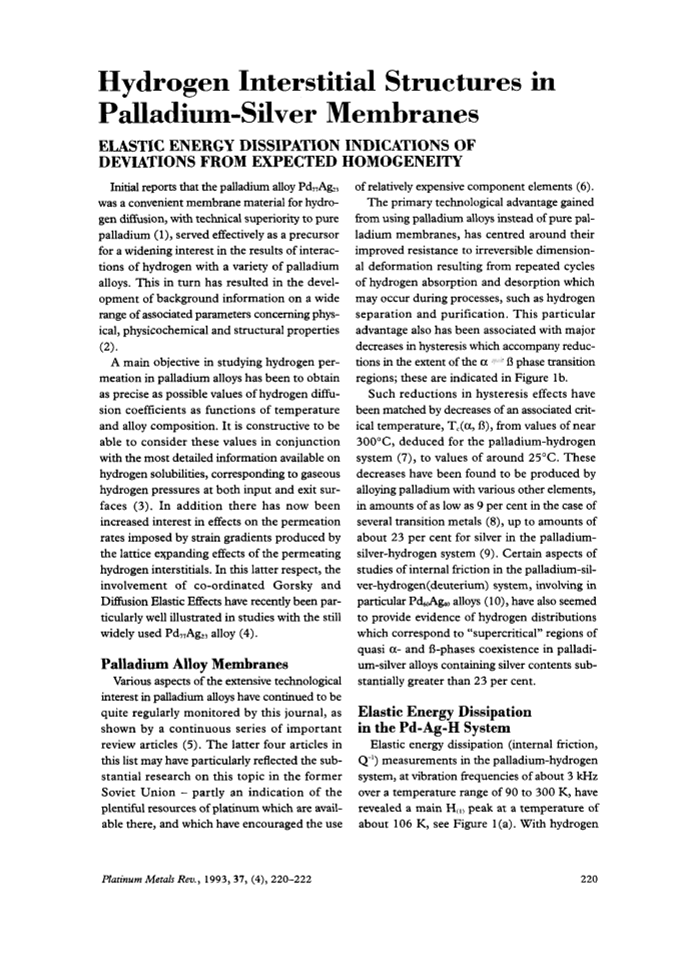



Hydrogen Interstitial Structures in Palladium-Silver Membranes, Page 1 of 1
< Previous page Next page > /docserver/preview/fulltext/pmr/37/4/pmr0037-0220-1.gif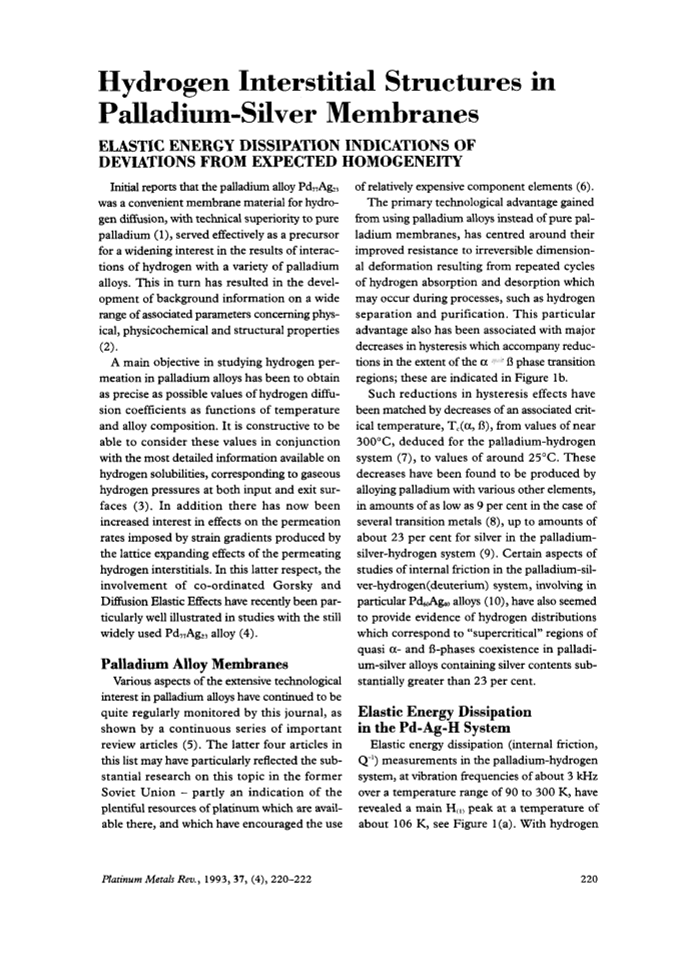
There is no abstract available.
© Johnson Matthey