-
oa Increasing the Resistance of Titanium to Non-Oxidising Acids
Influence Of Additions Of Platinum Metals
- Source: Platinum Metals Review, Volume 4, Issue 2, Apr 1960, p. 59 - 64
-
- 01 Jan 1960
Preview this article:
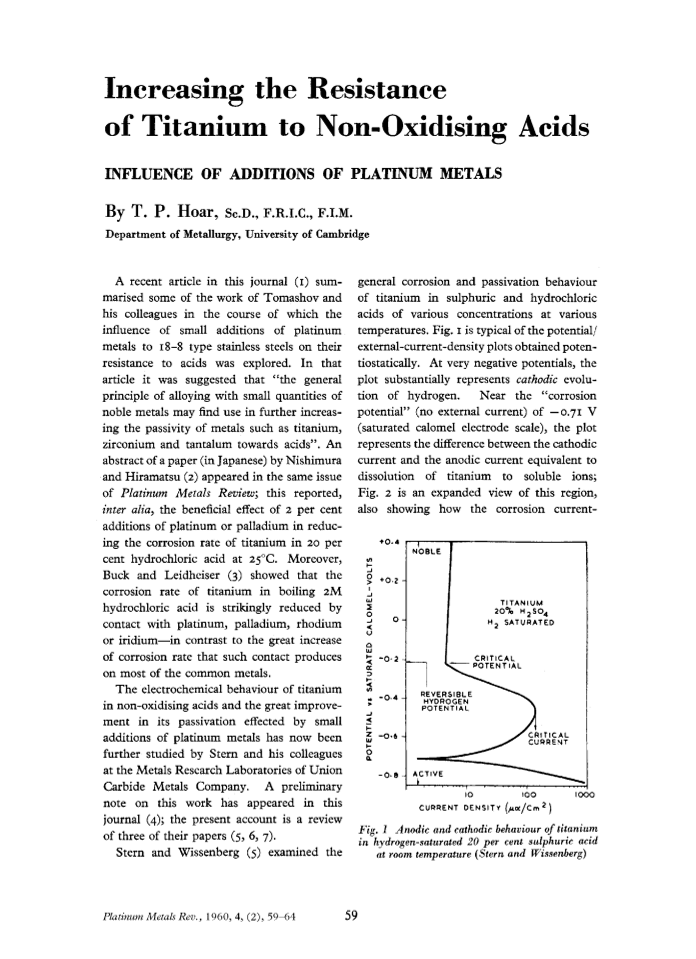



Increasing the Resistance of Titanium to Non-Oxidising Acids, Page 1 of 1
< Previous page Next page > /docserver/preview/fulltext/pmr/4/2/pmr0004-0059-1.gif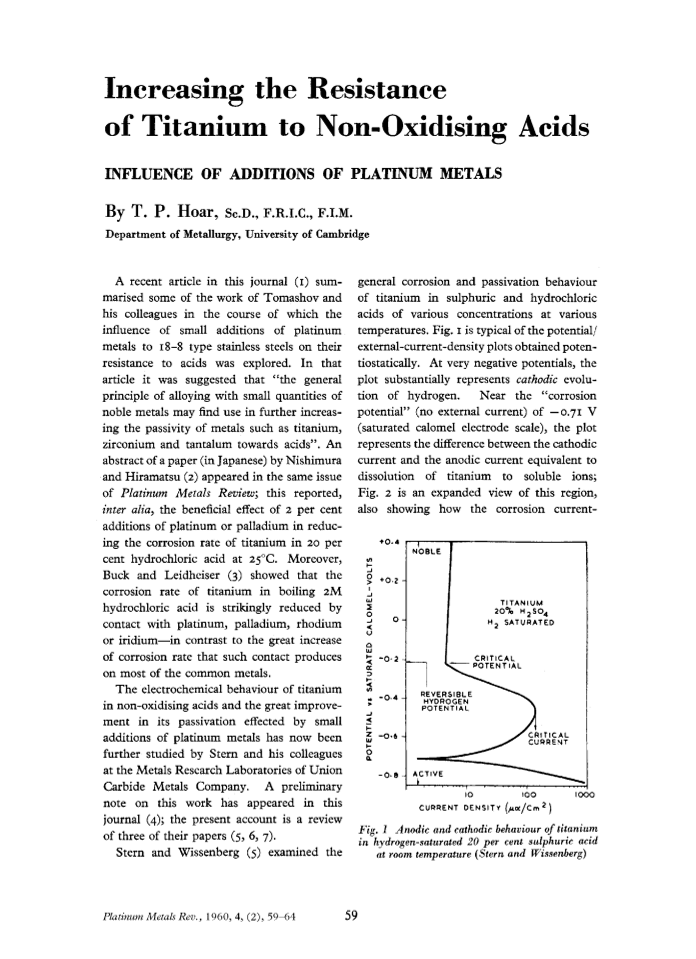
There is no abstract available.
© Johnson Matthey