-
oa Platinum Catalysts in Ammonia Oxidation
Operating Conditions in Fisons New Nitric Acid Plant
- Source: Platinum Metals Review, Volume 4, Issue 4, Oct 1960, p. 122 - 126
-
- 01 Jan 1960
Preview this article:
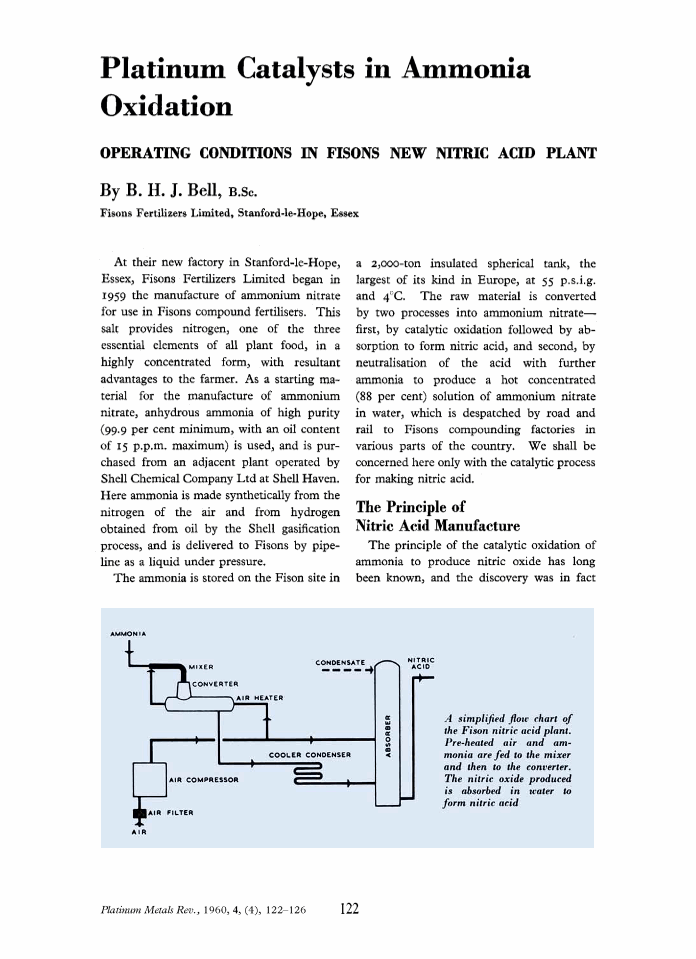



Platinum Catalysts in Ammonia Oxidation, Page 1 of 1
< Previous page Next page > /docserver/preview/fulltext/pmr/4/4/pmr0004-0122-1.gif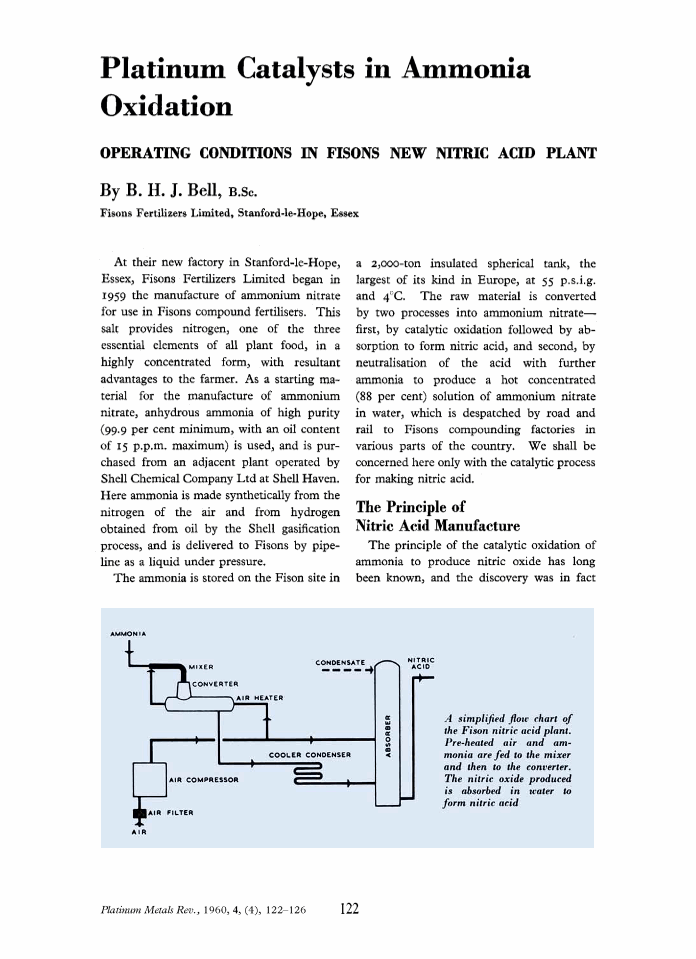
There is no abstract available.
© Johnson Matthey