-
oa Palladium-Mediated Heterogeneous Catalytic Hydrogenations
- Source: Platinum Metals Review, Volume 42, Issue 3, Jul 1998, p. 108 - 115
Preview this article:
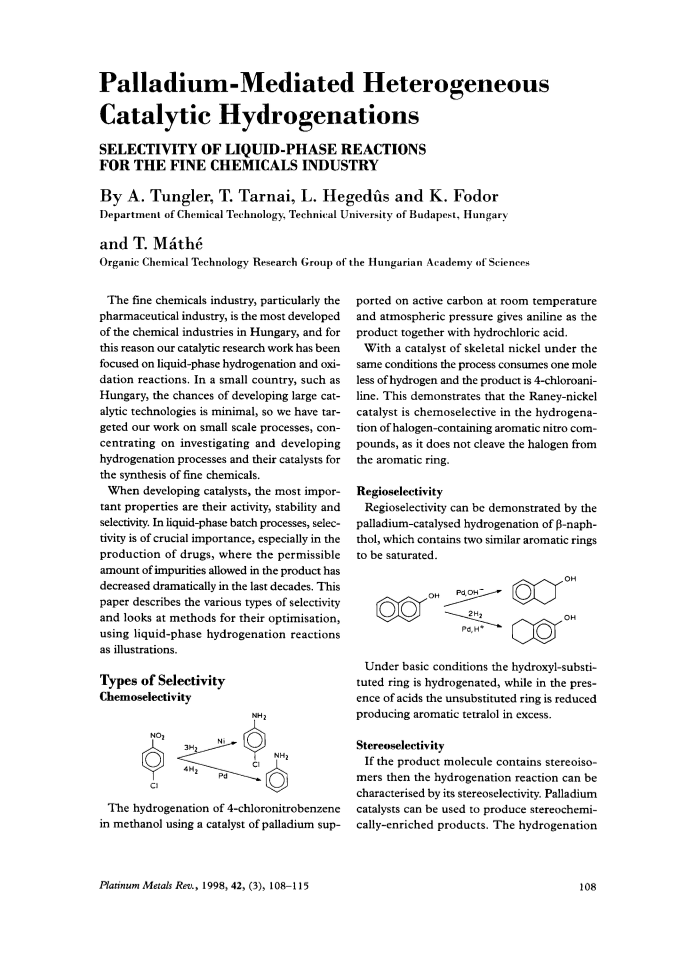



Palladium-Mediated Heterogeneous Catalytic Hydrogenations, Page 1 of 1
< Previous page Next page > /docserver/preview/fulltext/pmr/42/3/s7-1.gif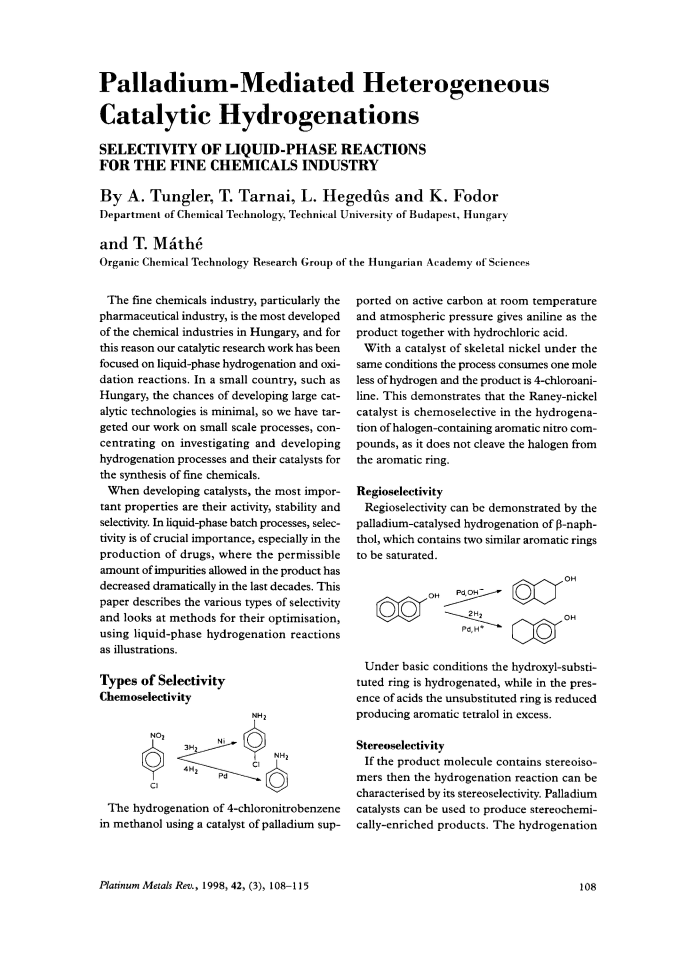
There is no abstract available.
© Johnson Matthey