-
oa Fuel Cells – Science and Technology 2004
SCIENTIFIC ADVANCES IN FUEL CELL SYSTEMS REPORTED IN MUNICH
- Source: Platinum Metals Review, Volume 49, Issue 1, Jan 2005, p. 16 - 20
-
- 01 Jan 2005
Preview this article:
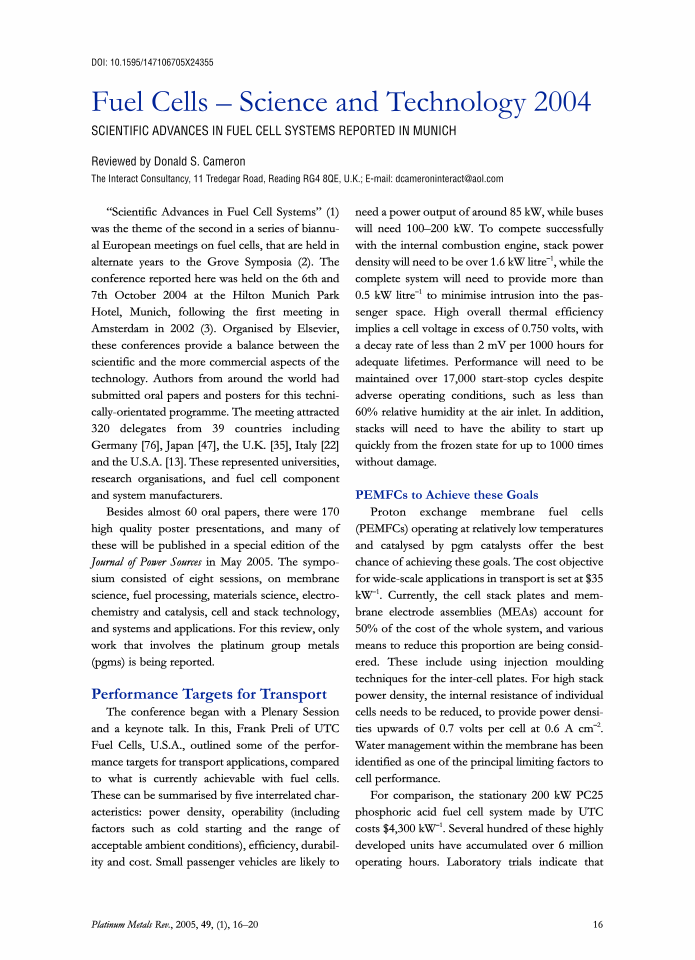



Fuel Cells – Science and Technology 2004, Page 1 of 1
< Previous page Next page > /docserver/preview/fulltext/pmr/49/1/PMR-49-1-Cameron-1.gif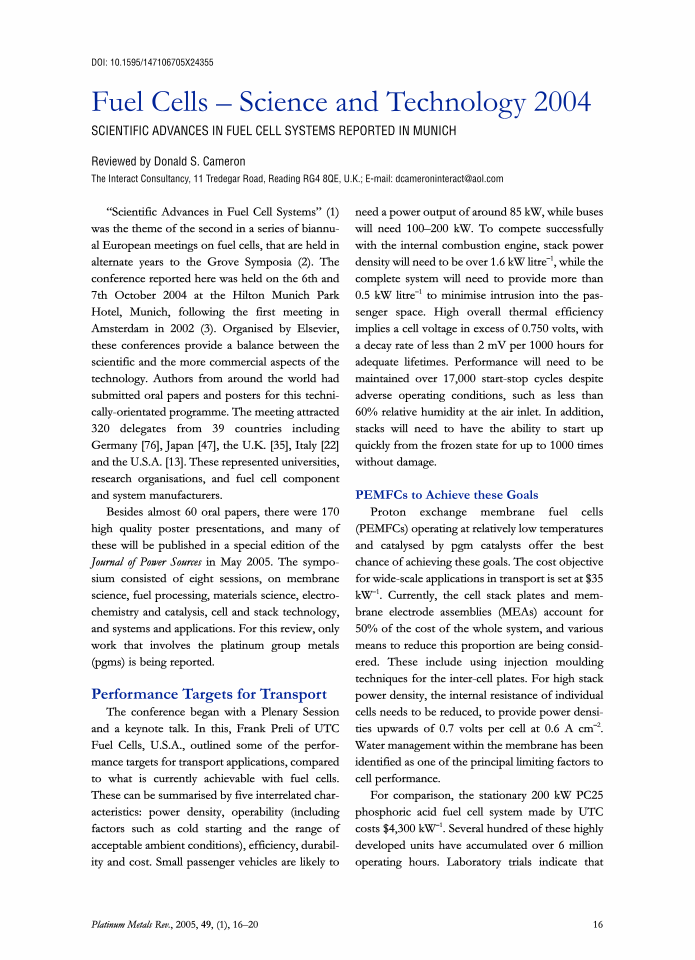
There is no abstract available.
© Johnson Matthey