-
oa The Ninth Grove Fuel Cell Symposium
BUILDING AND COMMERCIALISATION OF A FUEL CELL INDUSTRY – A PROGRESS REPORT
- Source: Platinum Metals Review, Volume 50, Issue 1, Jan 2006, p. 38 - 45
-
- 01 Jan 2006
Preview this article:
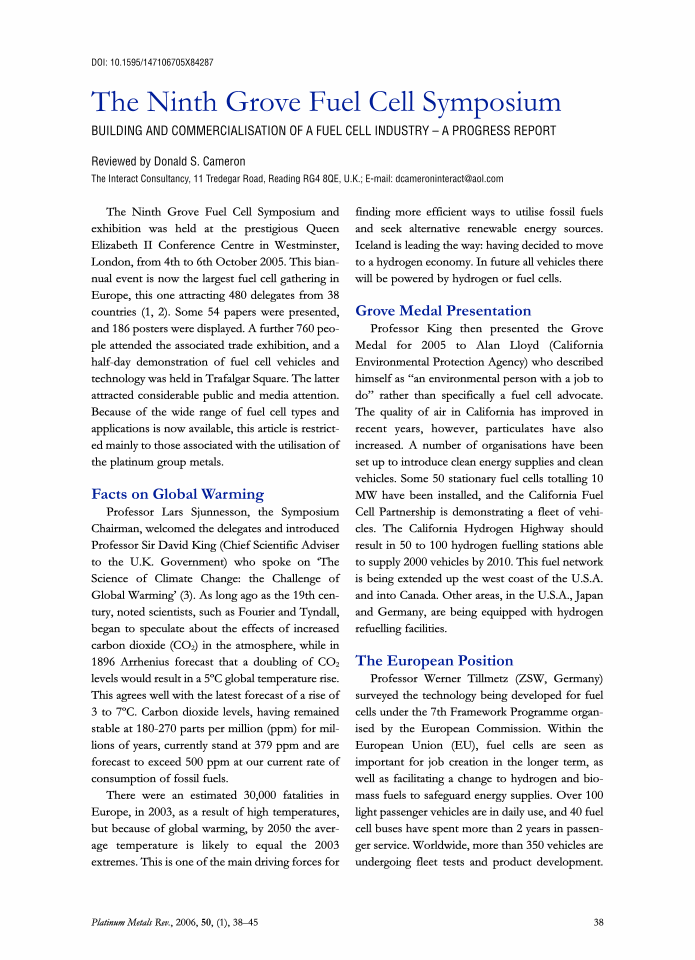



The Ninth Grove Fuel Cell Symposium, Page 1 of 1
< Previous page Next page > /docserver/preview/fulltext/pmr/50/1/PMR-50-1-Cameron-1.gif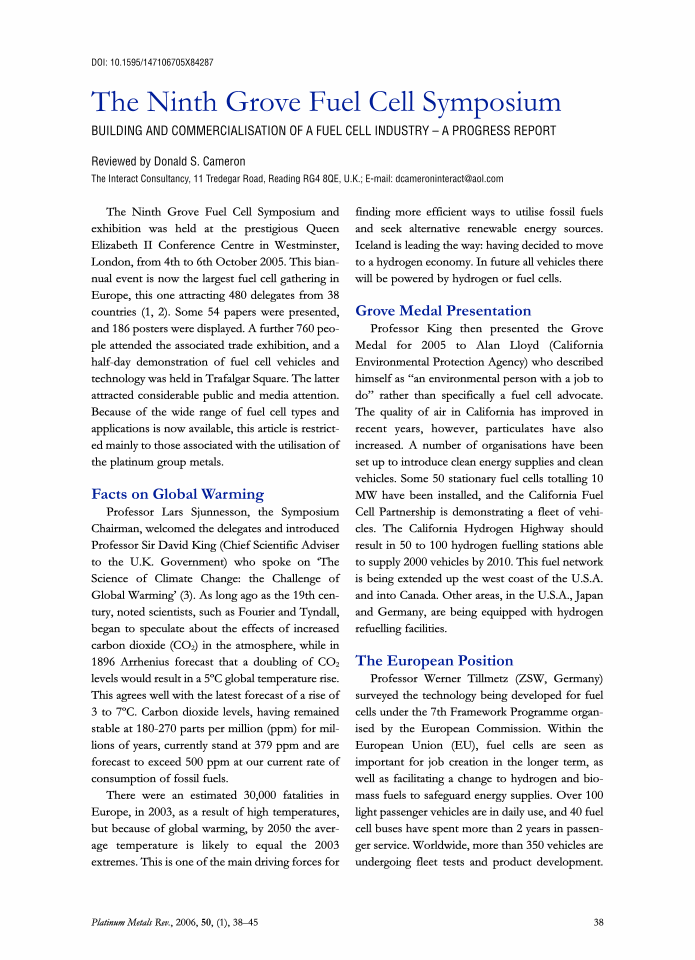
There is no abstract available.
© Johnson Matthey