-
oa “Nonporous Inorganic Membranes: for Chemical Processing”
EDITED BY ANTHONY F. SAMMELLS AND MICHAEL V. MUNDSCHAU (Eltron Research Inc, U.S.A.), Wiley-Vch, Weinheim, Germany, 2006, 291 pages, ISBN 978-3-527-31342-6, £80.00, ¢120.00
- Source: Platinum Metals Review, Volume 51, Issue 3, Jul 2007, p. 136 - 137
-
- 01 Jul 2007
Preview this article:
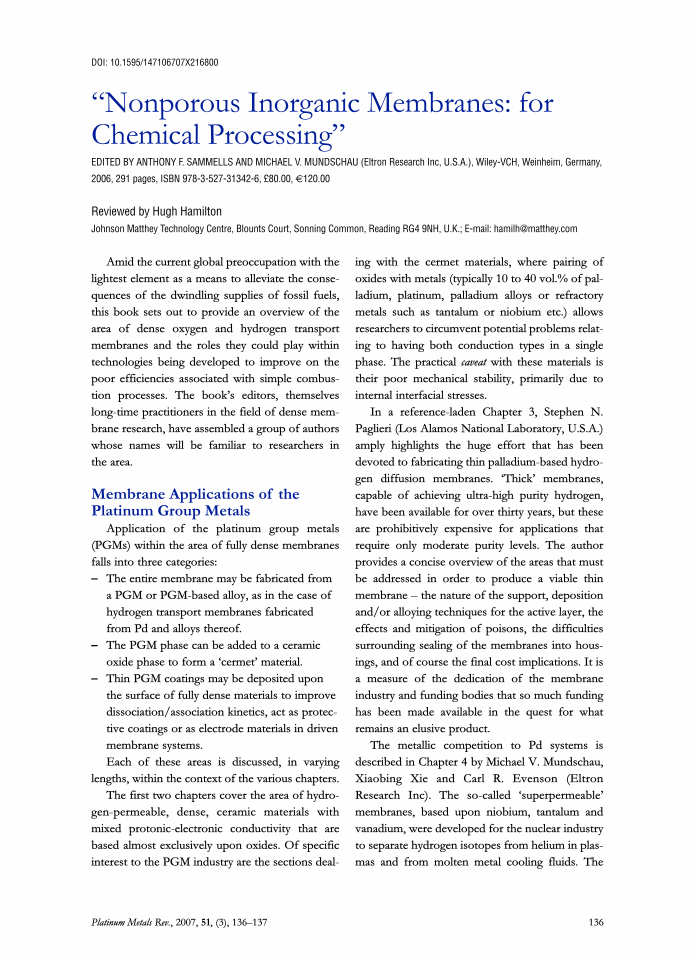



“Nonporous Inorganic Membranes: for Chemical Processing”, Page 1 of 1
< Previous page Next page > /docserver/preview/fulltext/pmr/51/3/PMR-51-3-Jul07-Hugh-1.gif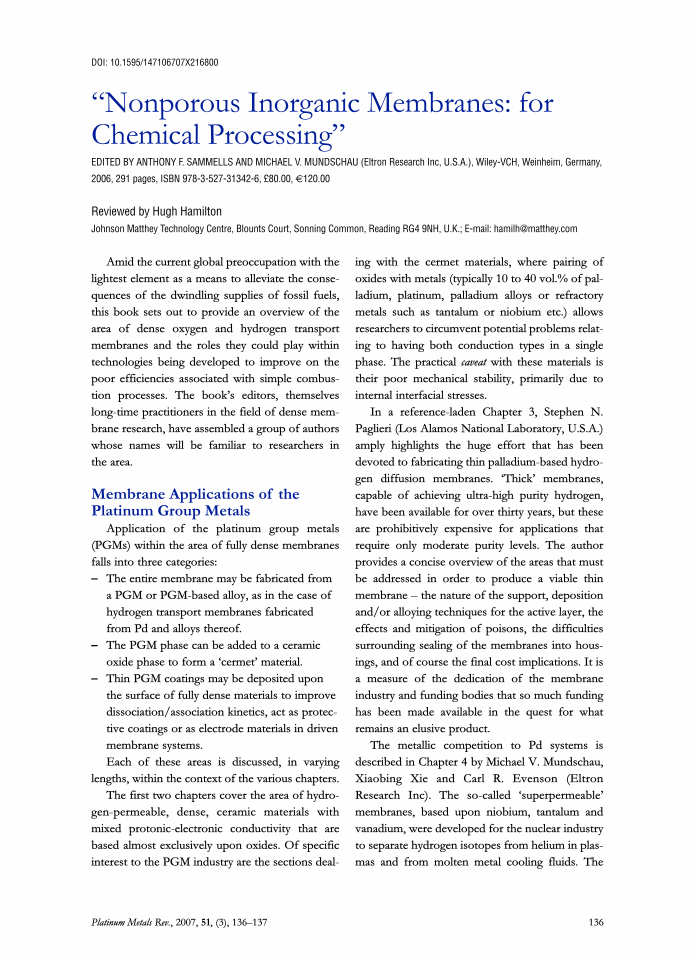
There is no abstract available.
© Johnson Matthey