-
oa “Combinatorial and High-Throughput Discovery and Optimization of Catalysts and Materials”
CRITICAL REVIEWS IN COMBINATORIAL CHEMISTRY, VOLUME 1
- Source: Platinum Metals Review, Volume 51, Issue 4, Oct 2007, p. 204 - 207
-
- 01 Jan 2016
Preview this article:
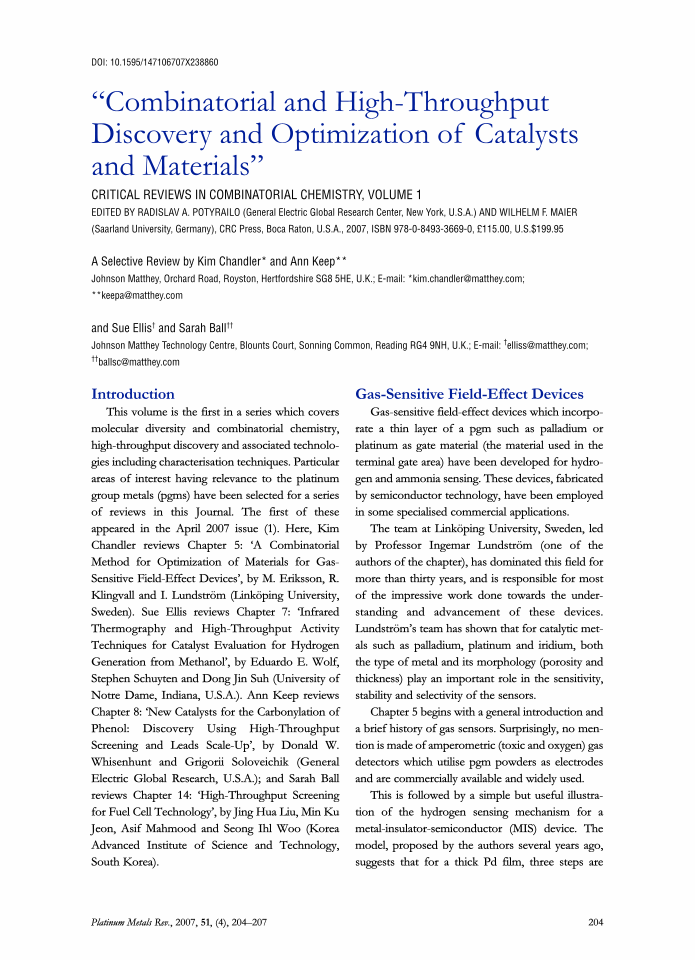



“Combinatorial and High-Throughput Discovery and Optimization of Catalysts and Materials”, Page 1 of 1
< Previous page Next page > /docserver/preview/fulltext/pmr/51/4/PMR-51-4-Chandler-1.gif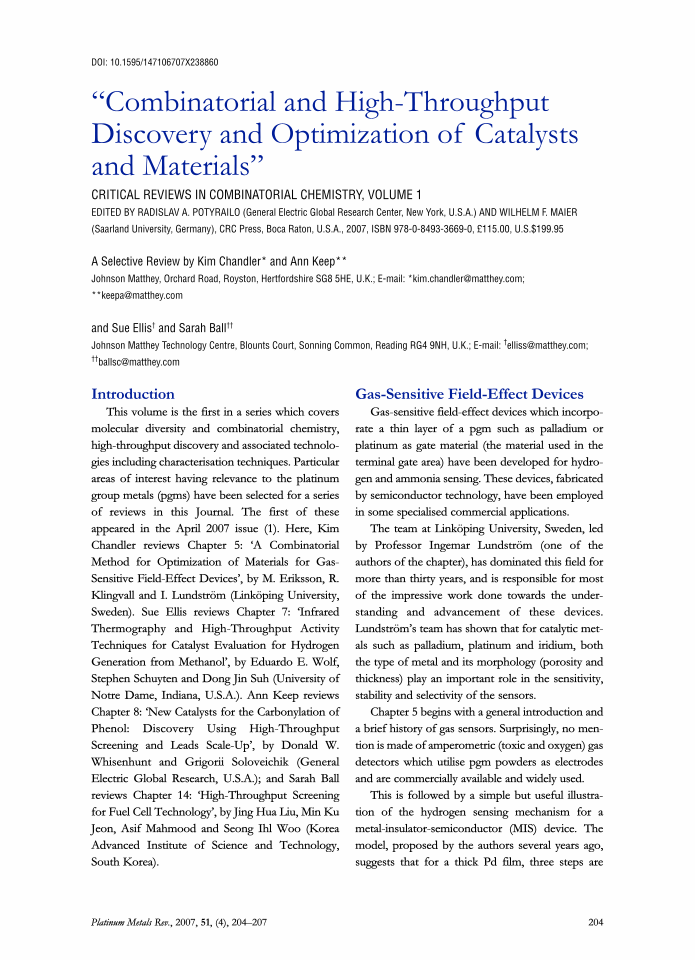
There is no abstract available.
© Johnson Matthey