-
oa Challenges in Catalysis for Pharmaceuticals and Fine Chemicals
PLATINUM GROUP METALS IN CATALYTIC PROCESSES
- Source: Platinum Metals Review, Volume 52, Issue 2, Apr 2008, p. 110 - 113
-
- 01 Jan 2008
Preview this article:
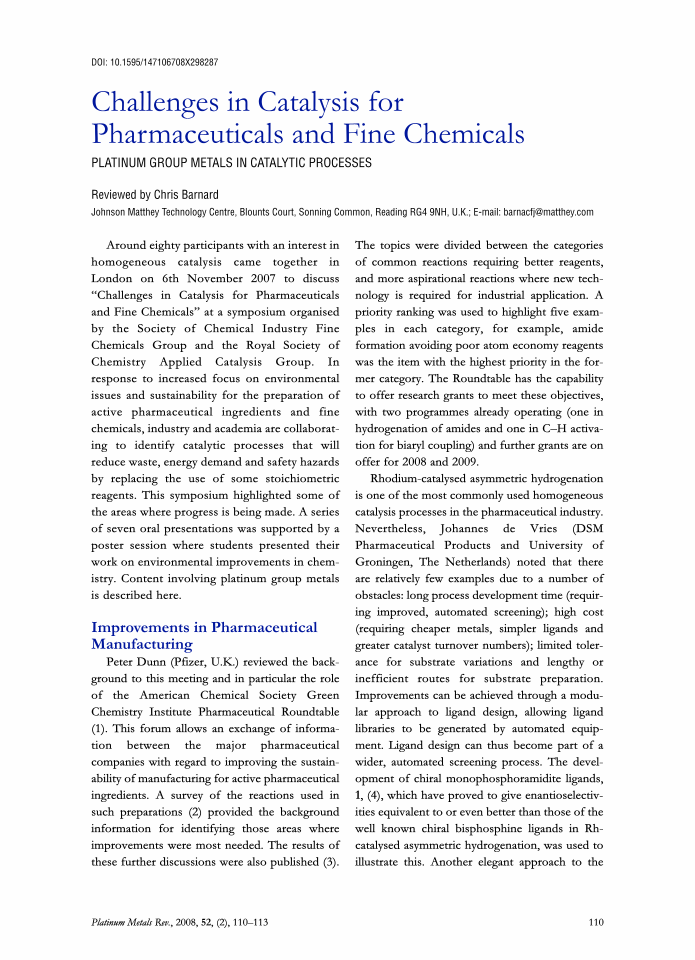



Challenges in Catalysis for Pharmaceuticals and Fine Chemicals, Page 1 of 1
< Previous page Next page > /docserver/preview/fulltext/pmr/52/2/PMR-52-2-Barnard-1.gif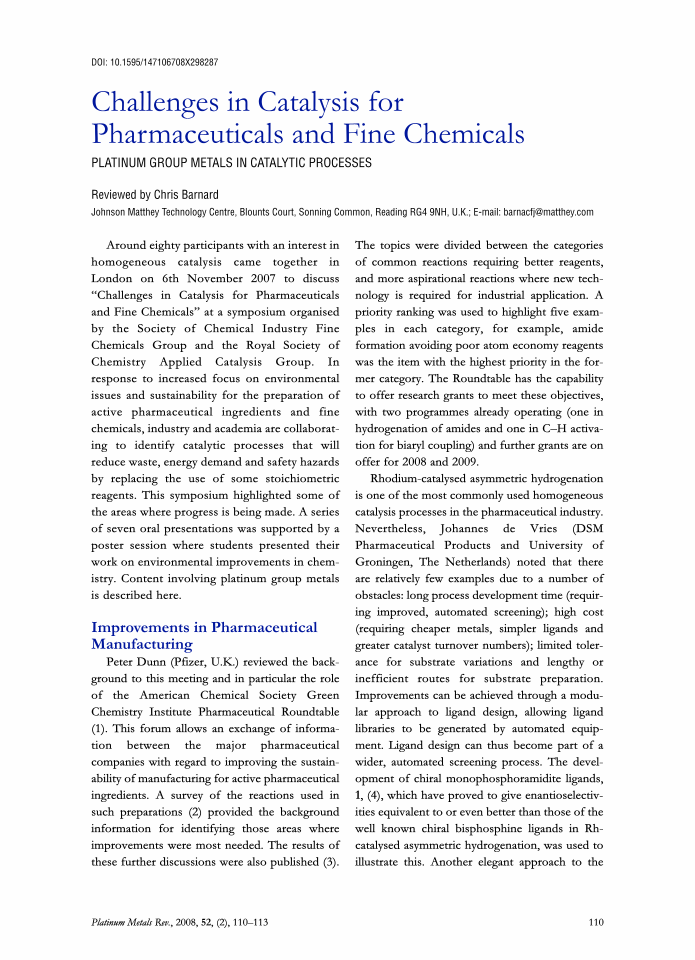
There is no abstract available.
© Johnson Matthey