-
oa "Carbons and Carbon Supported Catalysts in Hydroprocessing"
- Source: Platinum Metals Review, Volume 53, Issue 3, Jul 2009, p. 135 - 137
Preview this article:
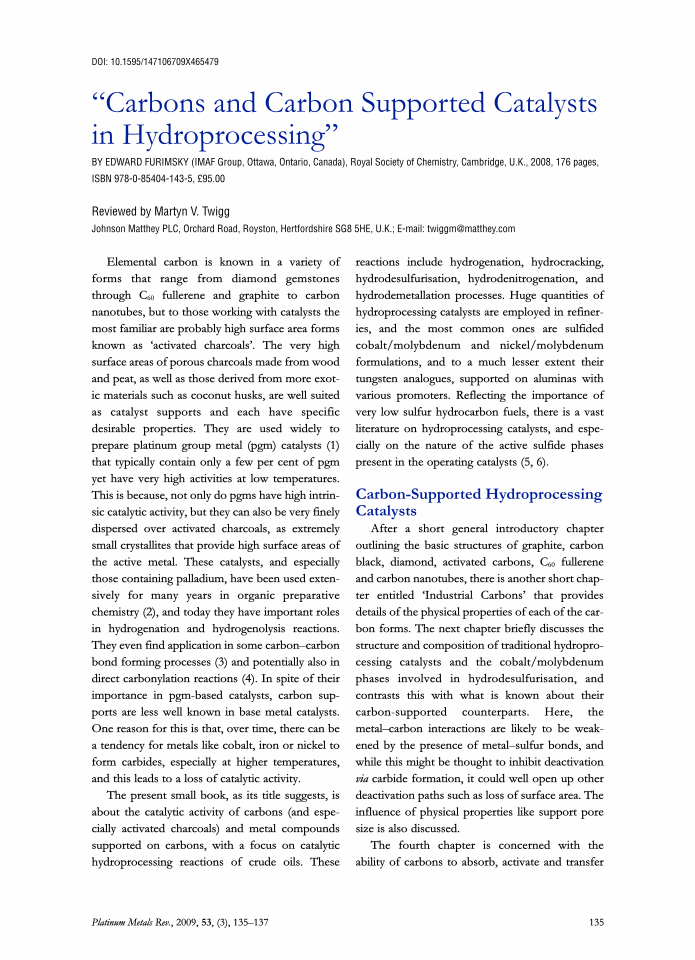



"Carbons and Carbon Supported Catalysts in Hydroprocessing", Page 1 of 1
< Previous page Next page > /docserver/preview/fulltext/pmr/53/3/s3-1.gif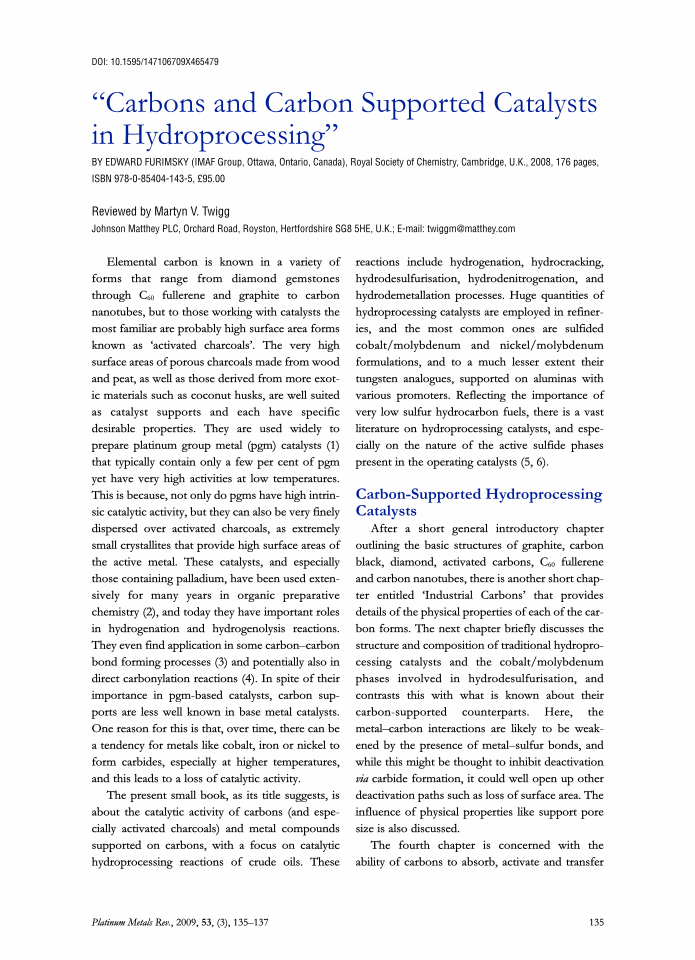
There is no abstract available.
© Johnson Matthey