-
oa 5th International Conference on Environmental Catalysis
- Source: Platinum Metals Review, Volume 53, Issue 3, Jul 2009, p. 164 - 171
Preview this article:
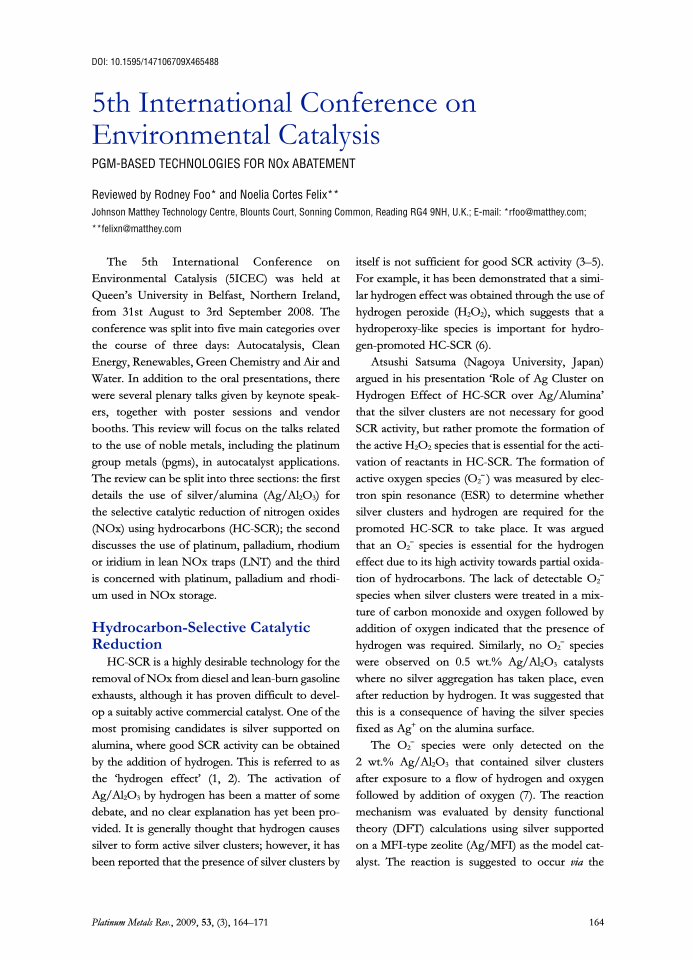



5th International Conference on Environmental Catalysis, Page 1 of 1
< Previous page Next page > /docserver/preview/fulltext/pmr/53/3/s7-1.gif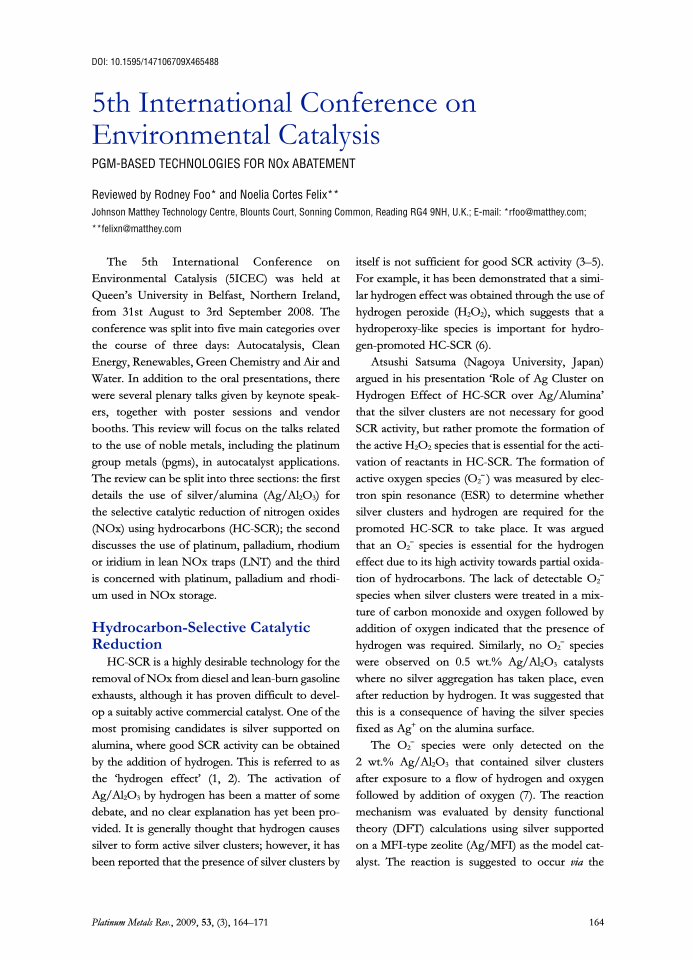
There is no abstract available.
© Johnson Matthey