-
oa FINAL ANALYSIS: Melting the PGMs: From Burning Glass to Laser Technology
- Source: Platinum Metals Review, Volume 54, Issue 2, Apr 2010, p. 133 - 135
-
- 01 Jan 2010
Preview this article:
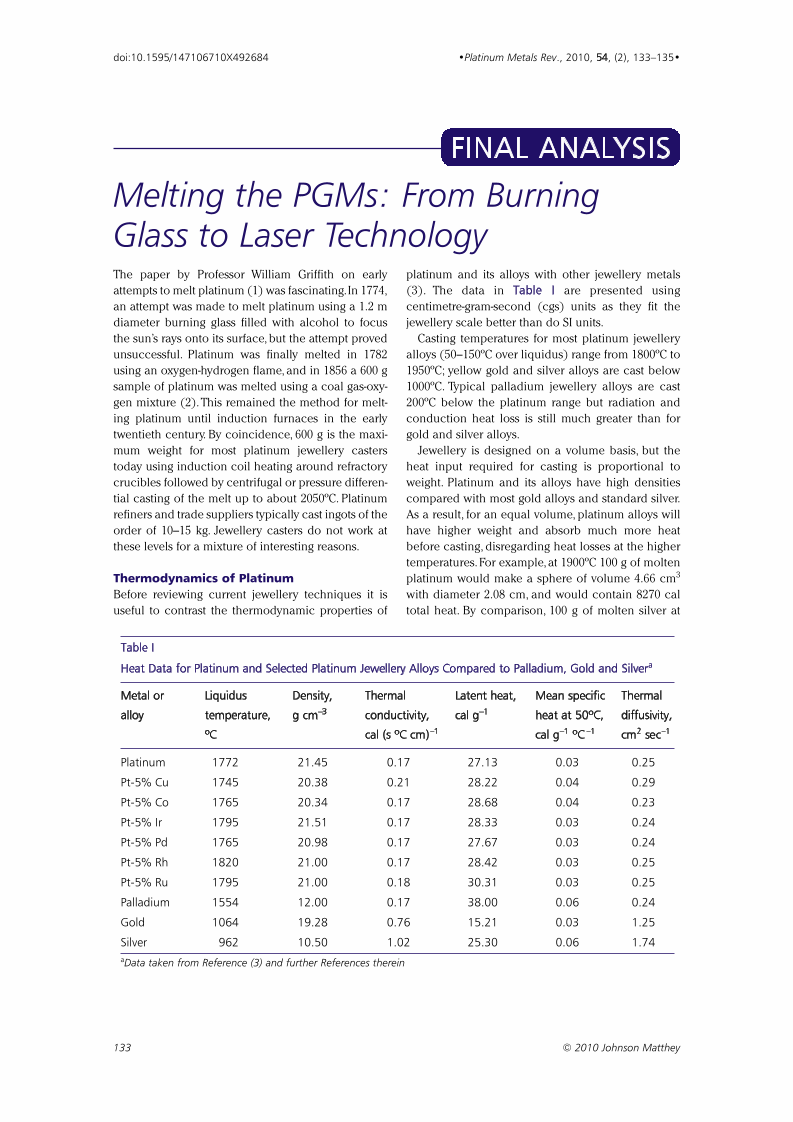



FINAL ANALYSIS: Melting the PGMs: From Burning Glass to Laser Technology, Page 1 of 1
< Previous page Next page > /docserver/preview/fulltext/pmr/54/2/PMR-54-2-Wright-1.gif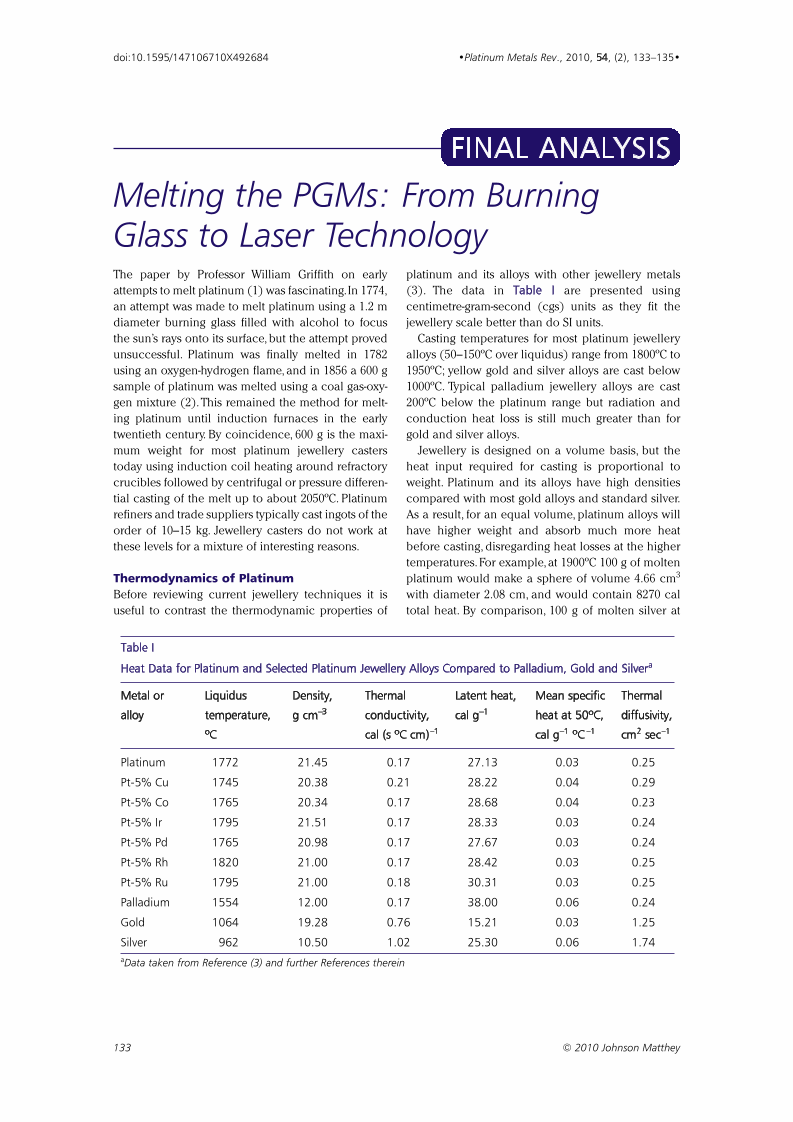
There is no abstract available.
© Johnson Matthey