-
oa Faraday Discussion 155: Artificial Photosynthesis
- Source: Platinum Metals Review, Volume 56, Issue 1, Jan 2012, p. 20 - 24
-
- 01 Jan 2012
Preview this article:
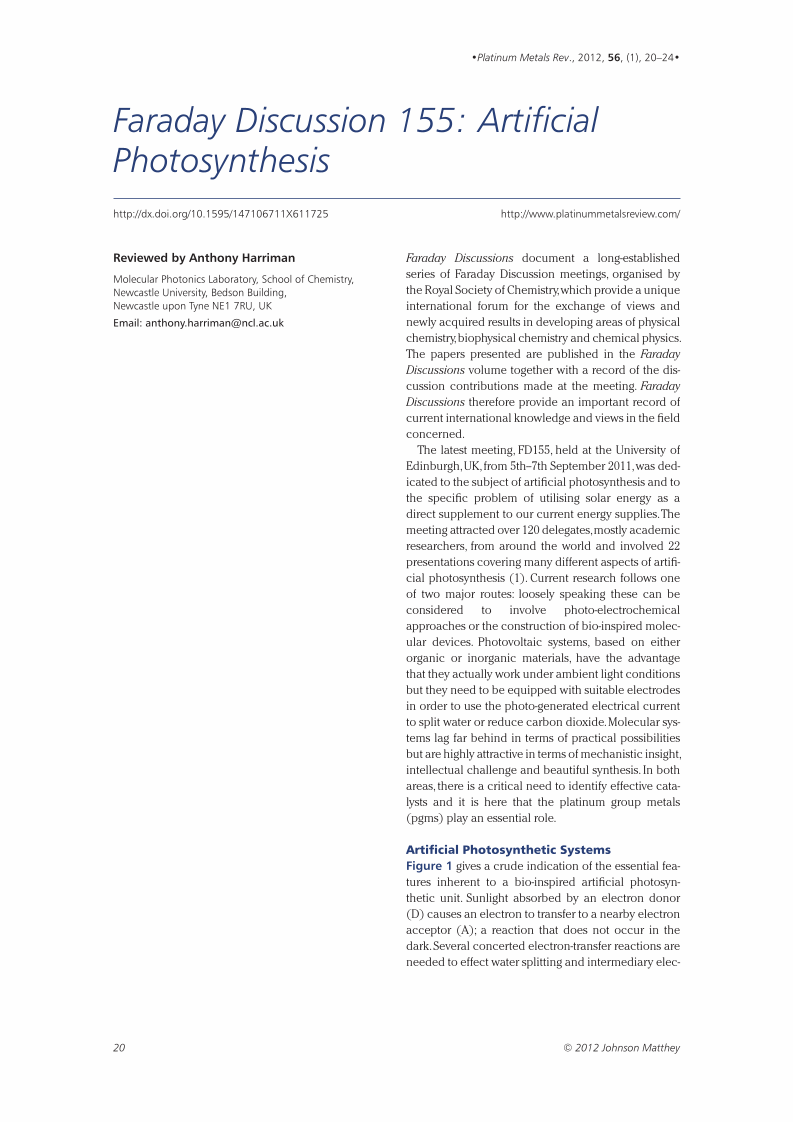



Faraday Discussion 155: Artificial Photosynthesis, Page 1 of 1
< Previous page Next page > /docserver/preview/fulltext/pmr/56/1/PMR-56-1-Harriman-1.gif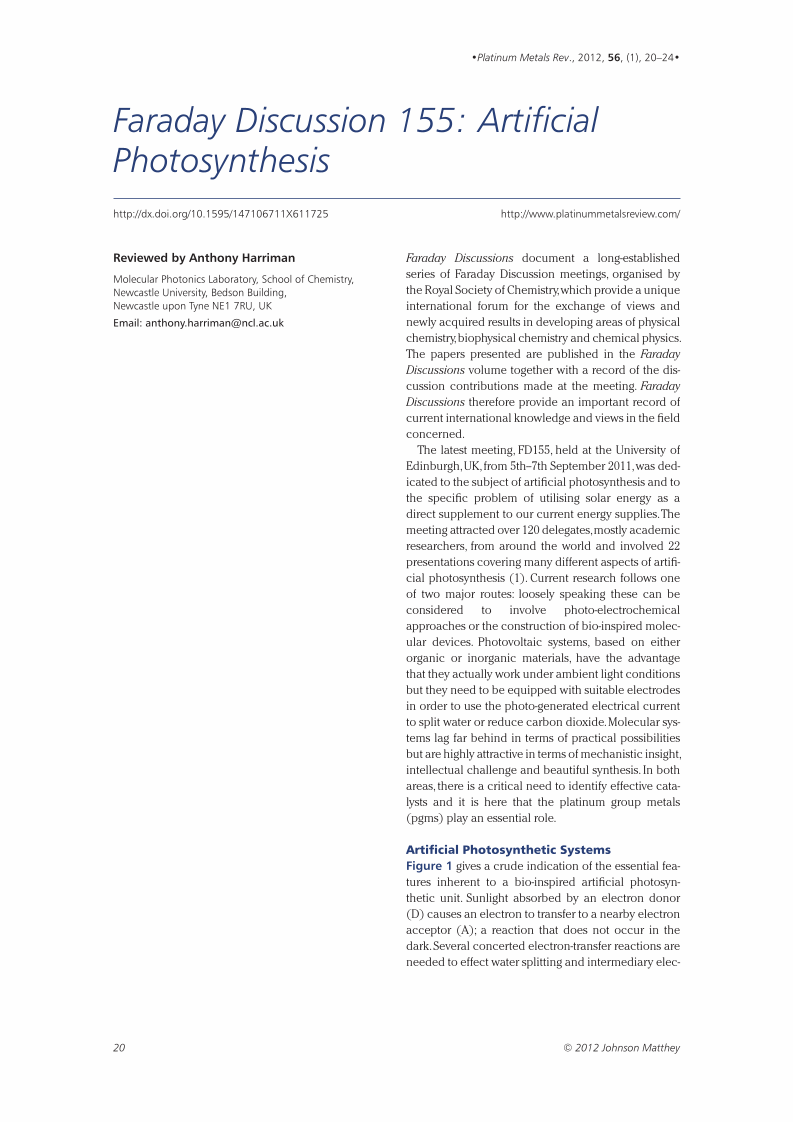
There is no abstract available.
© Johnson Matthey