-
oa Final Analysis: Biological or Chemical Catalysis?
- Source: Platinum Metals Review, Volume 56, Issue 2, Apr 2012, p. 140 - 142
-
- 01 Jan 2012
- Previous Article
- Table of Contents
- Next Article
Preview this article:
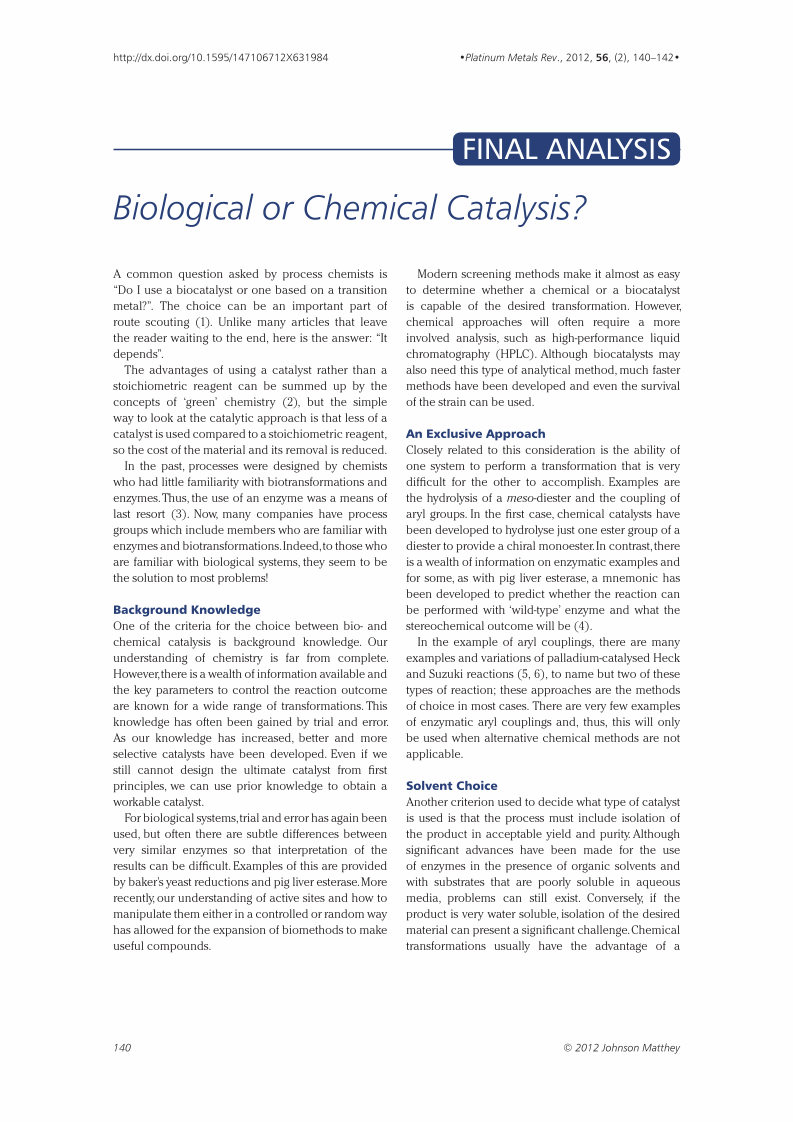



Final Analysis: Biological or Chemical Catalysis?, Page 1 of 1
< Previous page Next page > /docserver/preview/fulltext/pmr/56/2/PMR-56-2-Ager-1.gif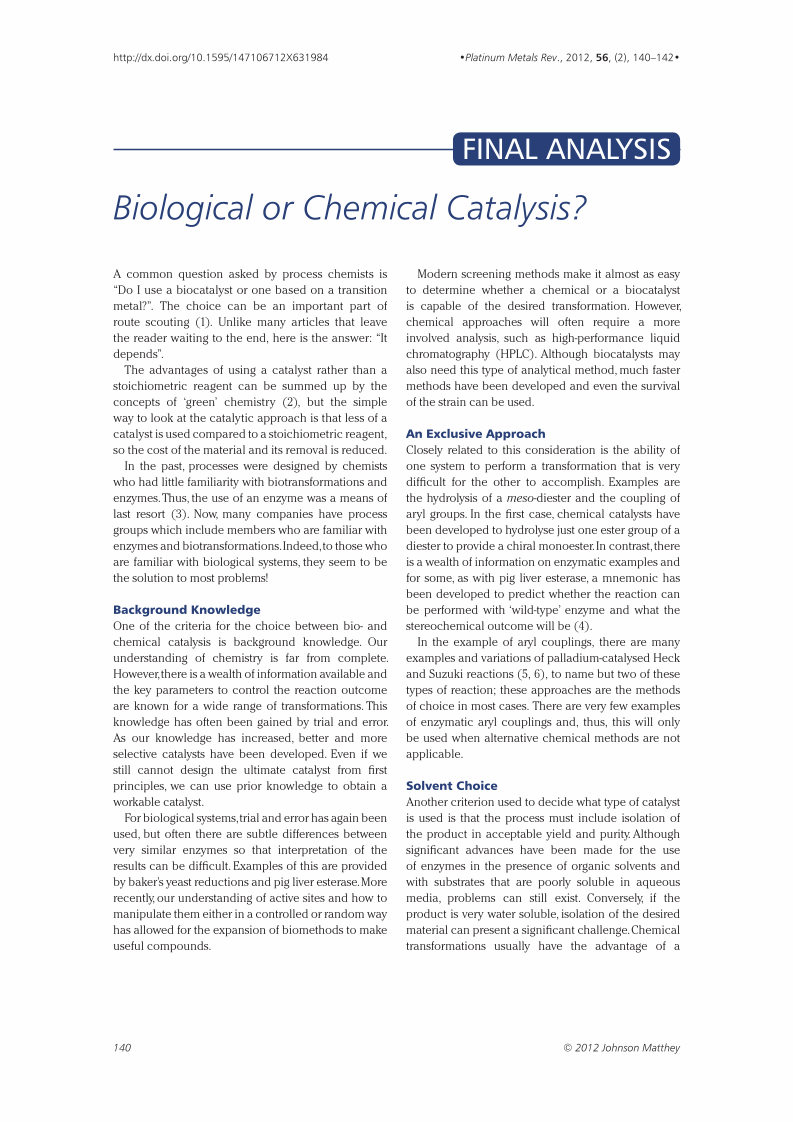
There is no abstract available.
© Johnson Matthey