-
oa “Total Synthesis of Bioactive Natural Products by Palladium-Catalyzed Domino Cyclization of Allenes and Related Compounds”
By Shinsuke Inuki (Kyoto University, Japan), Springer Theses, Springer, Tokyo, Japan, 2012, 106 pages, ISBN: 978-4-431-54042-7, £90.00, €106.95, US$129.00
- Source: Platinum Metals Review, Volume 56, Issue 3, Aug 2012, p. 194 - 199
-
- 01 Jan 2012
Preview this article:
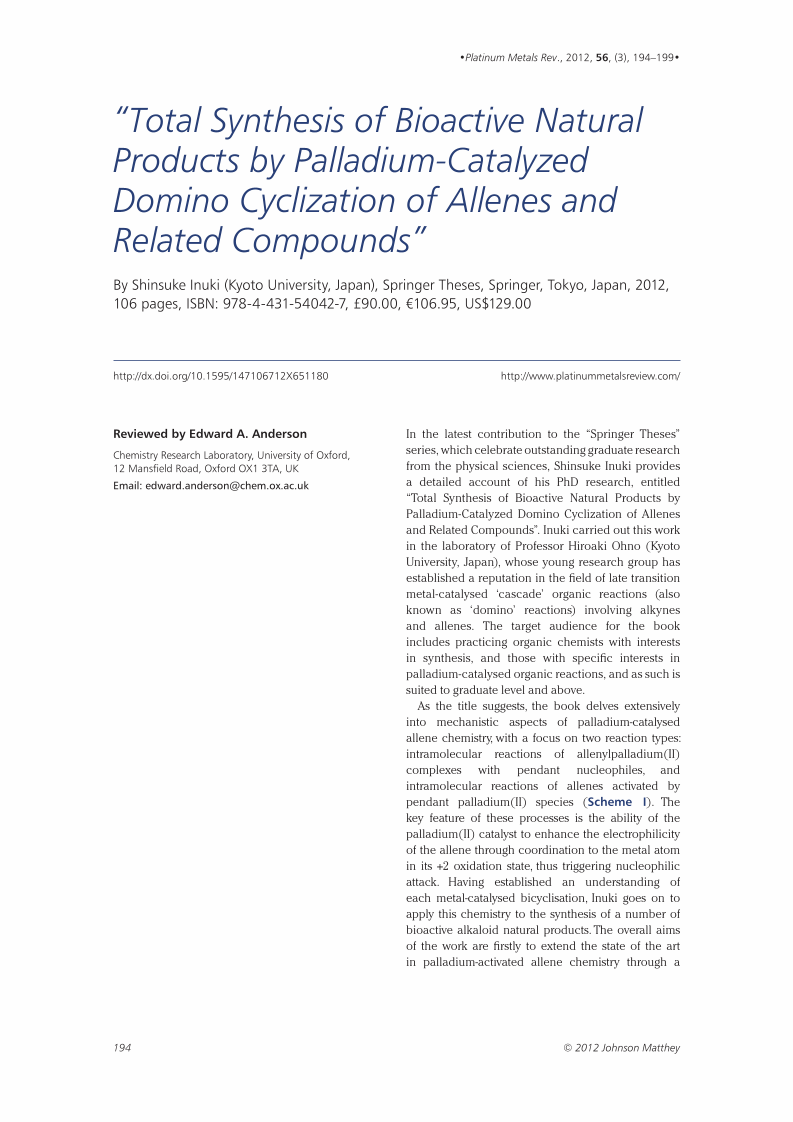



“Total Synthesis of Bioactive Natural Products by Palladium-Catalyzed Domino Cyclization of Allenes and Related Compounds”, Page 1 of 1
< Previous page Next page > /docserver/preview/fulltext/pmr/56/3/PMR-56-03-Anderson-1.gif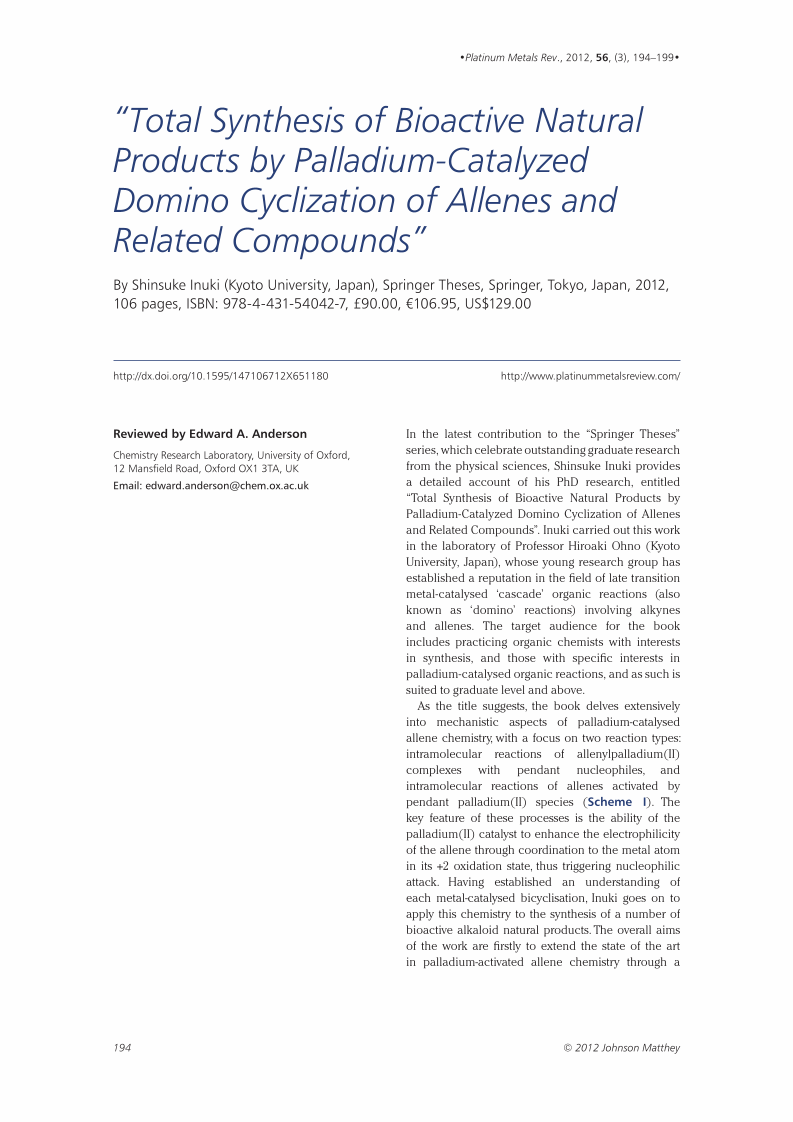
There is no abstract available.
© Johnson Matthey