-
oa Heterogeneous Catalytic Hydrogenation
Platinum group metals as hydrogenation catalysts in a two-day course
- Source: Platinum Metals Review, Volume 56, Issue 4, Oct 2012, p. 236 - 241
-
- 01 Jan 2012
Preview this article:
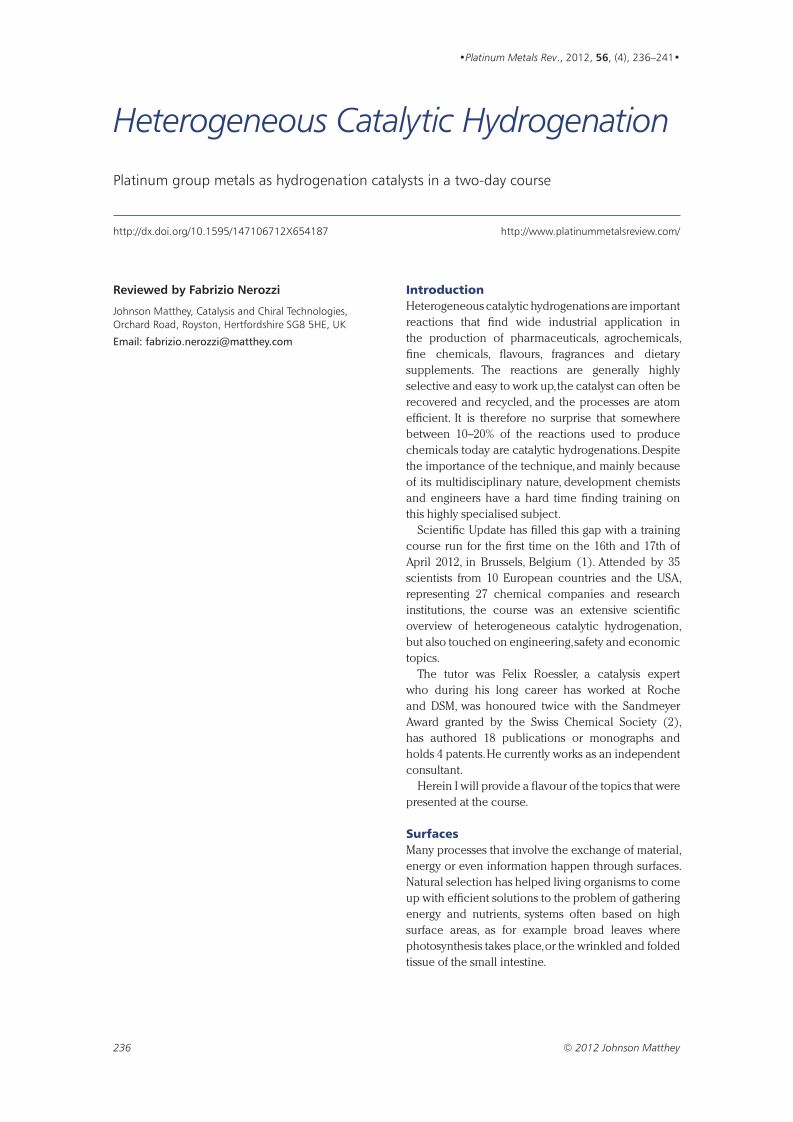



Heterogeneous Catalytic Hydrogenation, Page 1 of 1
< Previous page Next page > /docserver/preview/fulltext/pmr/56/4/PMR-56-4-Nerrozzi-1.gif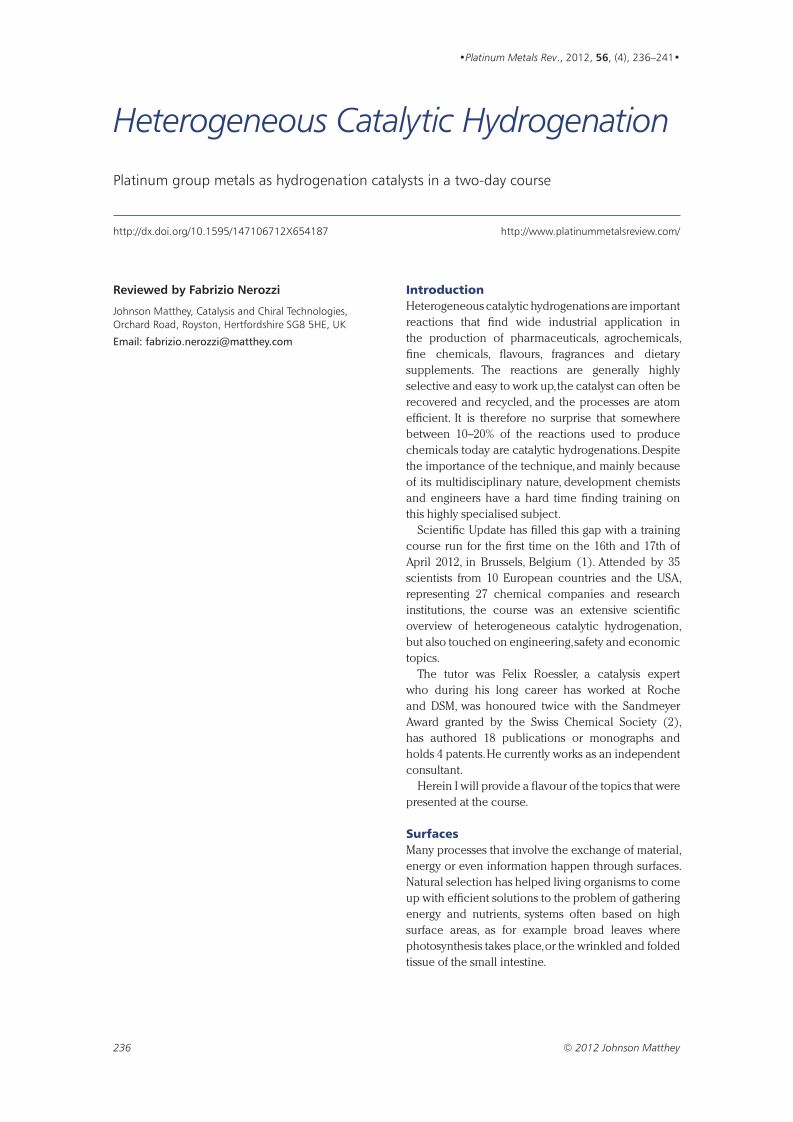
There is no abstract available.
© Johnson Matthey