-
oa The 3rd CARISMA International Conference on Medium and High Temperature Proton Exchange Membrane Fuel Cells
Three approaches to better platinum catalysts at biannual conference
- Source: Platinum Metals Review, Volume 57, Issue 3, Jul 2013, p. 173 - 176
-
- 01 Jan 2013
Preview this article:
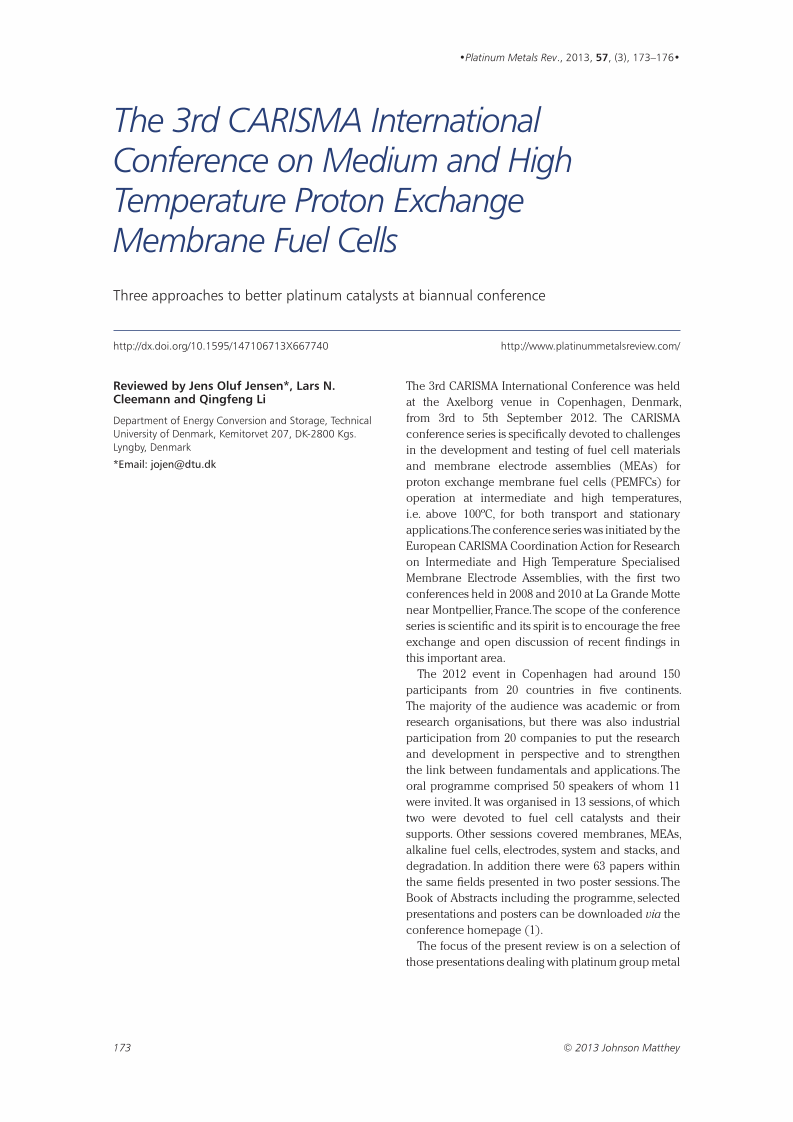



The 3rd CARISMA International Conference on Medium and High Temperature Proton Exchange Membrane Fuel Cells, Page 1 of 1
< Previous page Next page > /docserver/preview/fulltext/pmr/57/3/PMR-57-03-Jensen-1.gif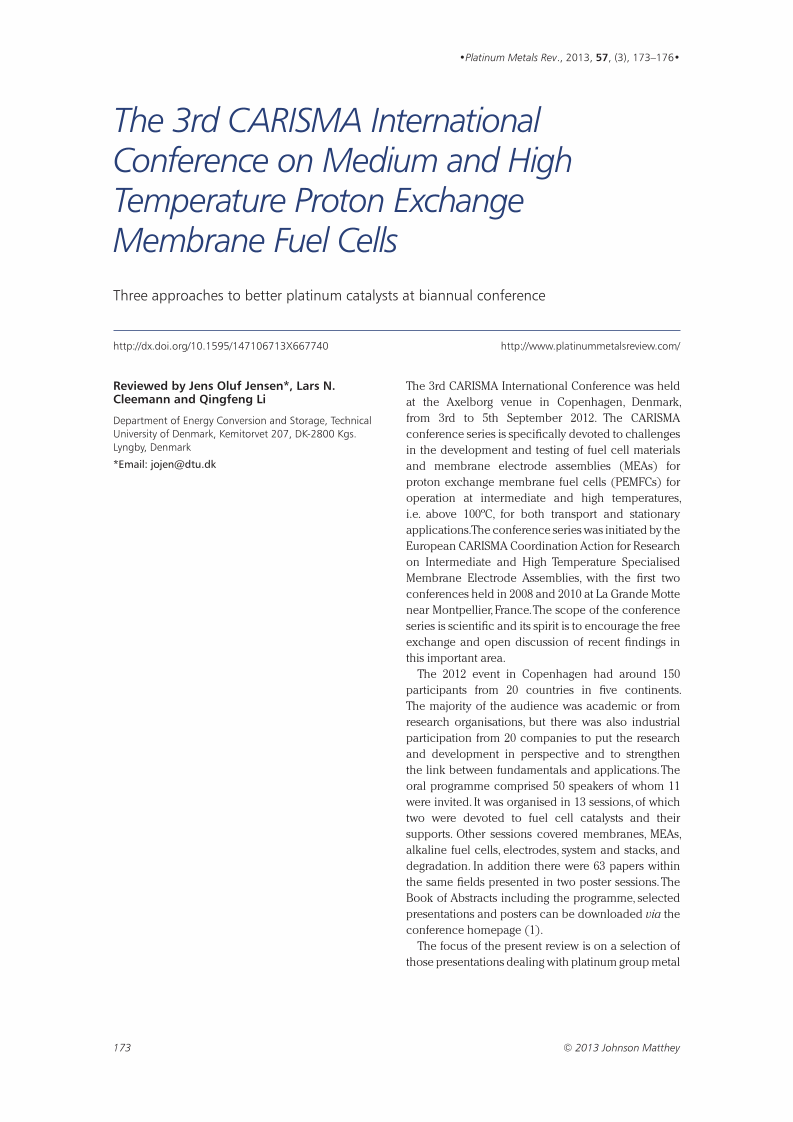
There is no abstract available.
© Johnson Matthey