-
oa “Catalysts for Alcohol-Fuelled Direct Oxidation Fuel Cells”
- Source: Platinum Metals Review, Volume 57, Issue 4, Oct 2013, p. 297 - 301
Preview this article:
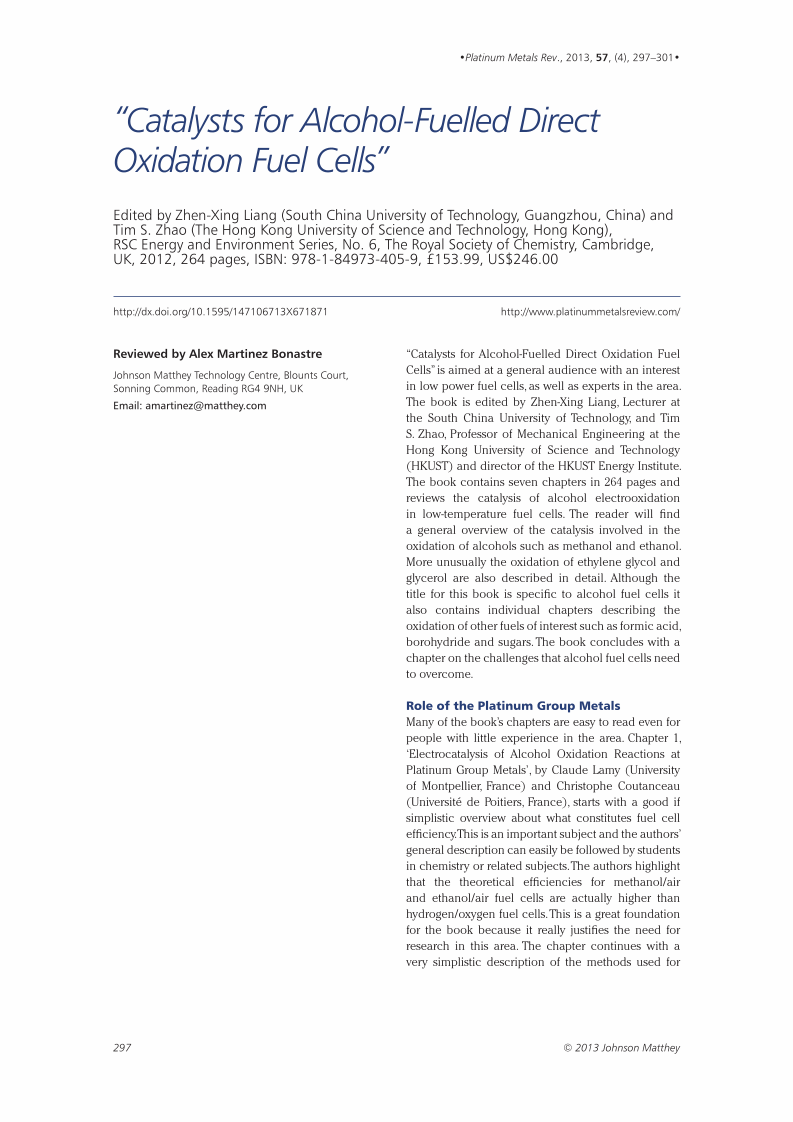



“Catalysts for Alcohol-Fuelled Direct Oxidation Fuel Cells”, Page 1 of 1
< Previous page Next page > /docserver/preview/fulltext/pmr/57/4/s6-1.gif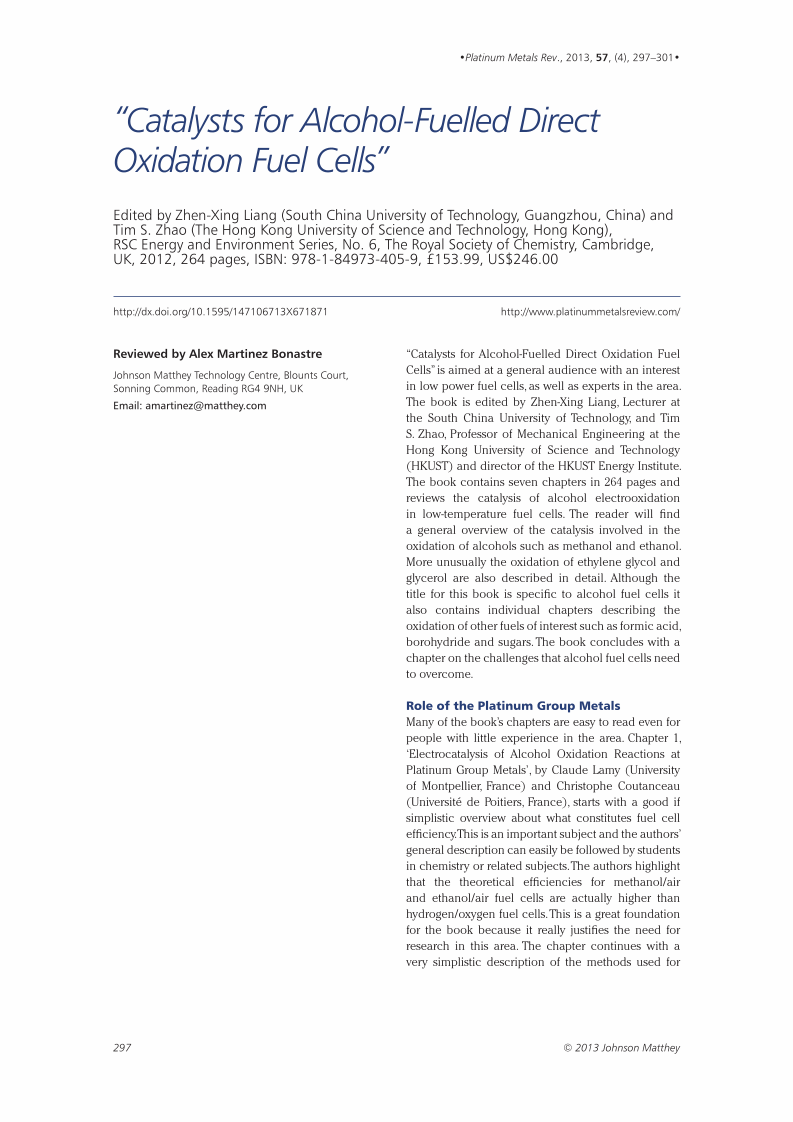
There is no abstract available.
© Johnson Matthey