-
oa FINAL ANALYSIS: Platinum Group Metal Catalysts for the Development of New Processes to Biorenewables
- Source: Platinum Metals Review, Volume 57, Issue 4, Oct 2013, p. 322 - 325
Preview this article:
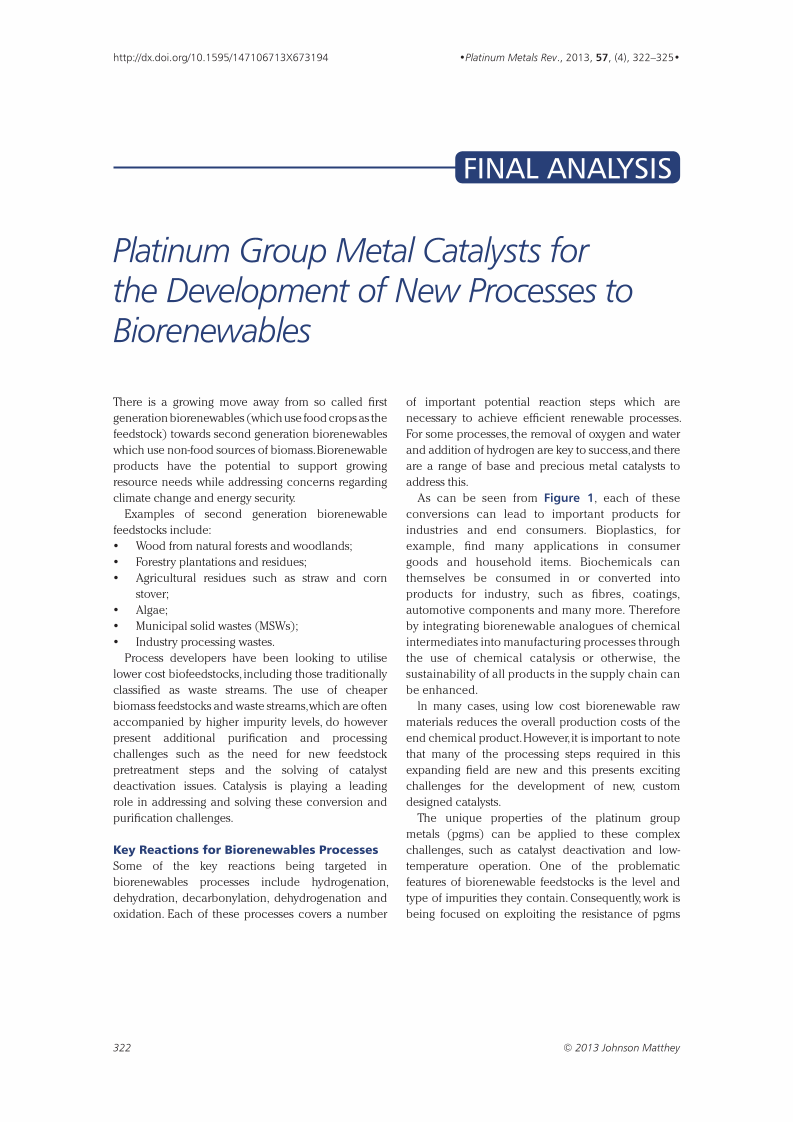



FINAL ANALYSIS: Platinum Group Metal Catalysts for the Development of New Processes to Biorenewables, Page 1 of 1
< Previous page Next page > /docserver/preview/fulltext/pmr/57/4/s13-1.gif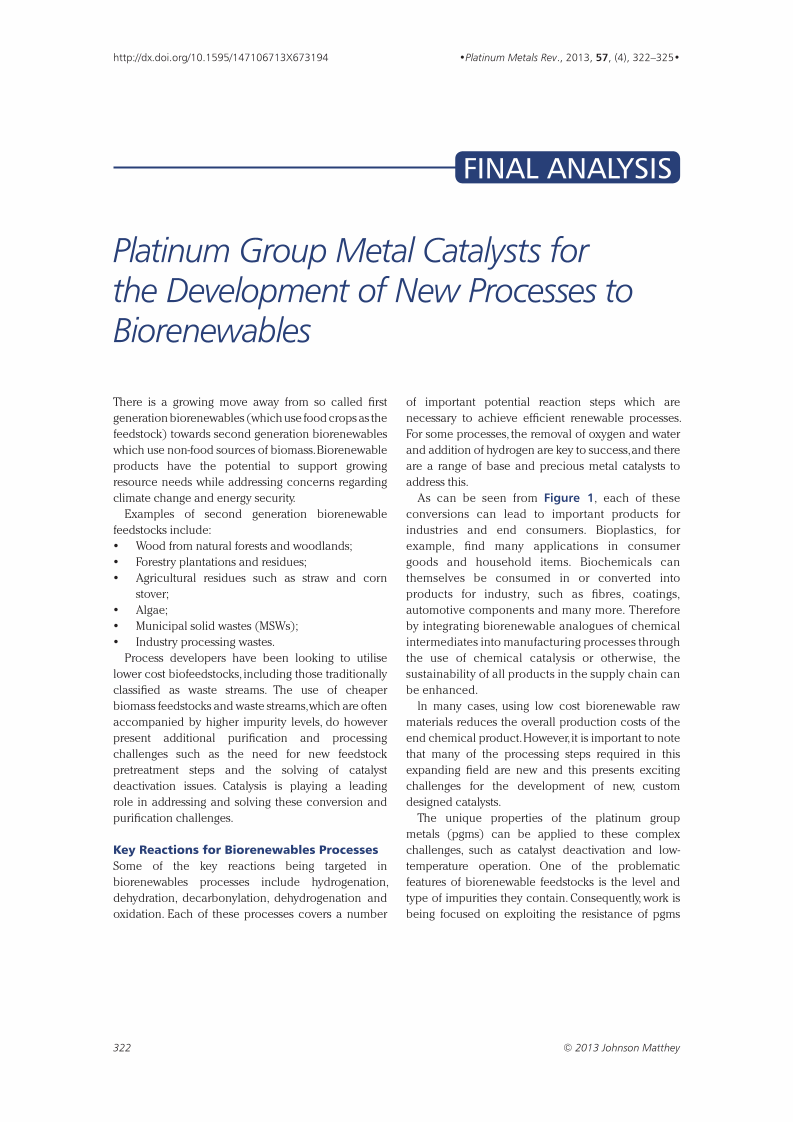
There is no abstract available.
© Johnson Matthey