-
oa Platinum Metals Predominate as Electrocatalysts in Fuel Cells
Papers at London and Brussels Conferences
- Source: Platinum Metals Review, Volume 9, Issue 4, Oct 1965, p. 119 - 121
-
- 01 Oct 1965
Preview this article:
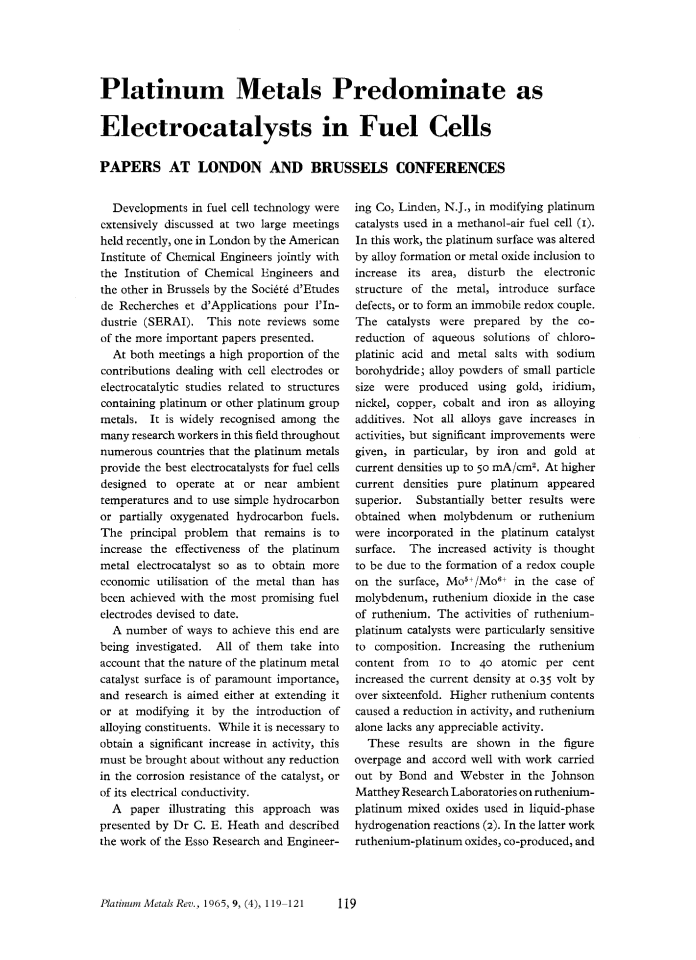



Platinum Metals Predominate as Electrocatalysts in Fuel Cells, Page 1 of 1
< Previous page Next page > /docserver/preview/fulltext/pmr/9/4/pmr0009-0119-1.gif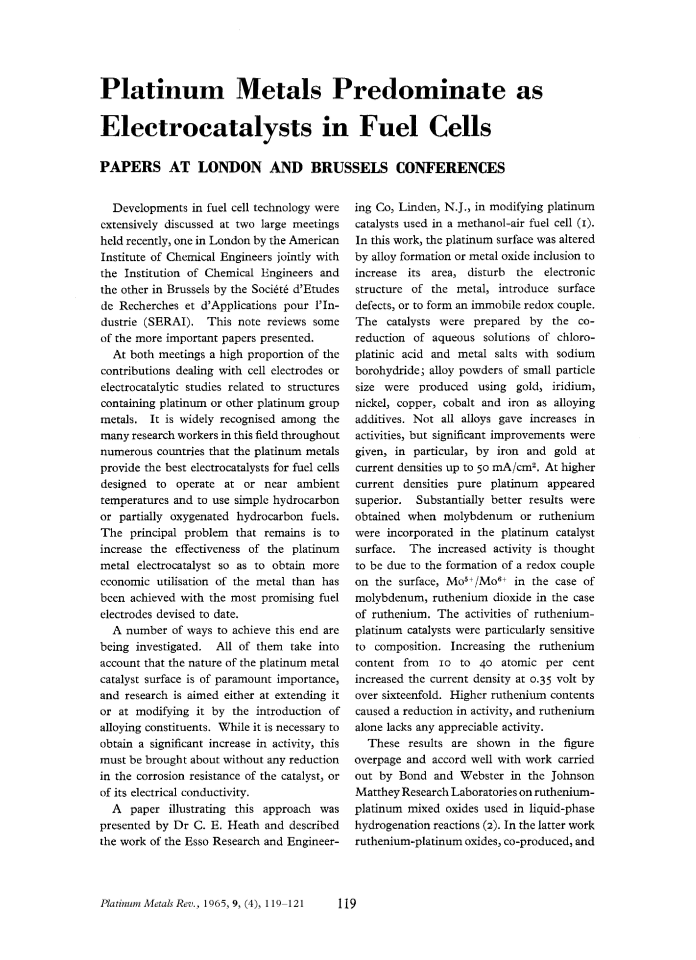
There is no abstract available.
© Johnson Matthey