-
oa Flow-Through Catalysts for Diesel Engine Emissions Control
Platinum Coated Monoliths Reduce Particulates
- Source: Platinum Metals Review, Volume 35, Issue 4, Oct 1991, p. 178 - 187
-
- 01 Jan 1991
Preview this article:
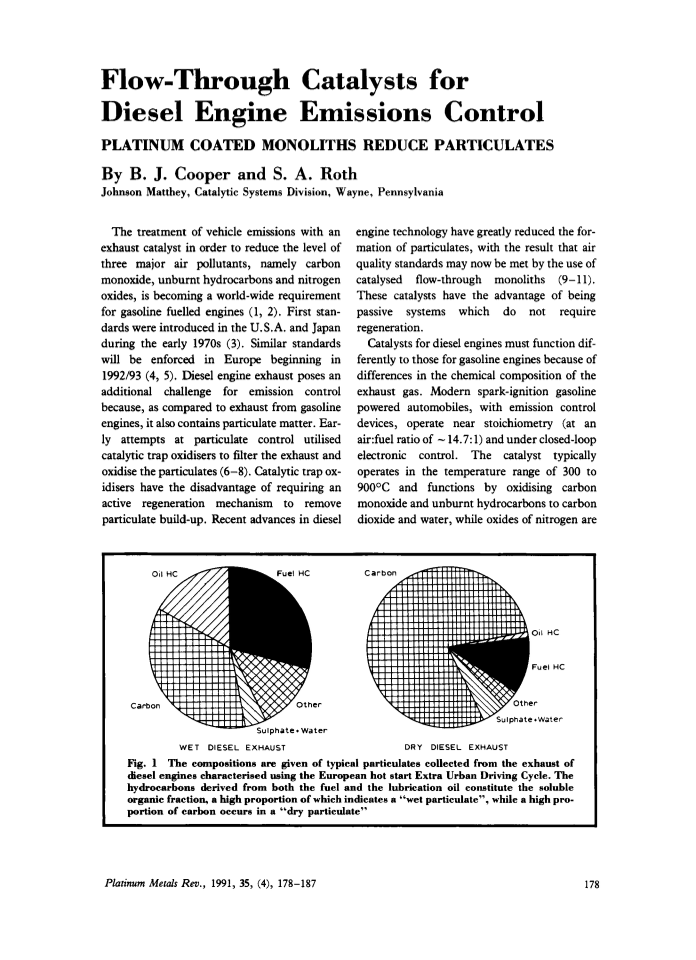



Flow-Through Catalysts for Diesel Engine Emissions Control, Page 1 of 1
< Previous page Next page > /docserver/preview/fulltext/pmr/35/4/pmr0035-0178-1.gif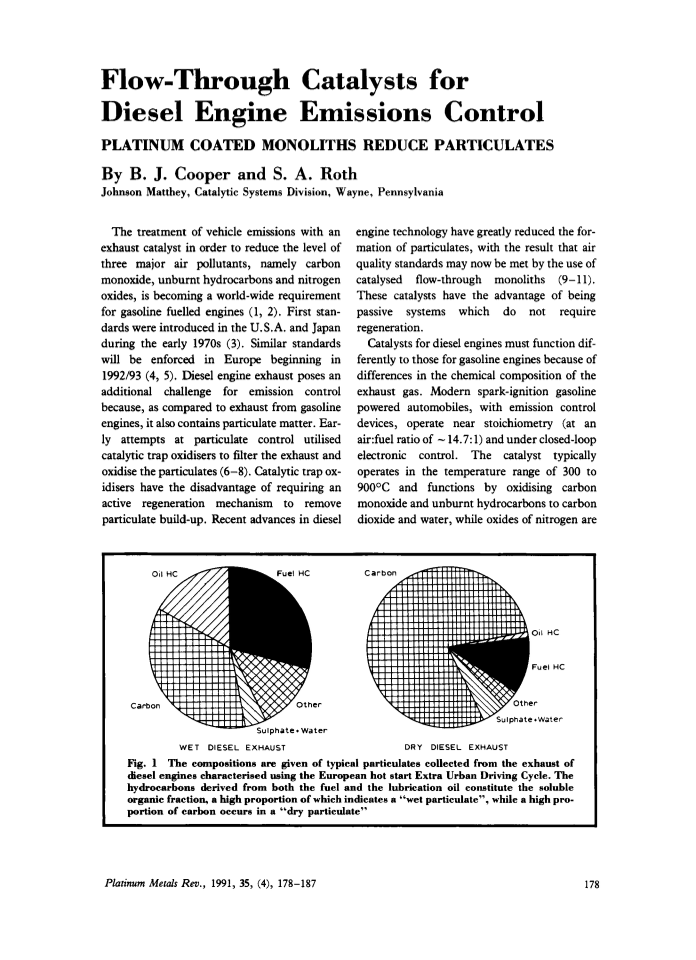
There is no abstract available.
© Johnson Matthey