-
oa Second Grove Fuel Cell Symposium
Platinum-Bearing Fuel Cells Emerge as Initial Choices for Commercialisation
- Source: Platinum Metals Review, Volume 35, Issue 4, Oct 1991, p. 209 - 221
-
- 01 Jan 1991
Preview this article:
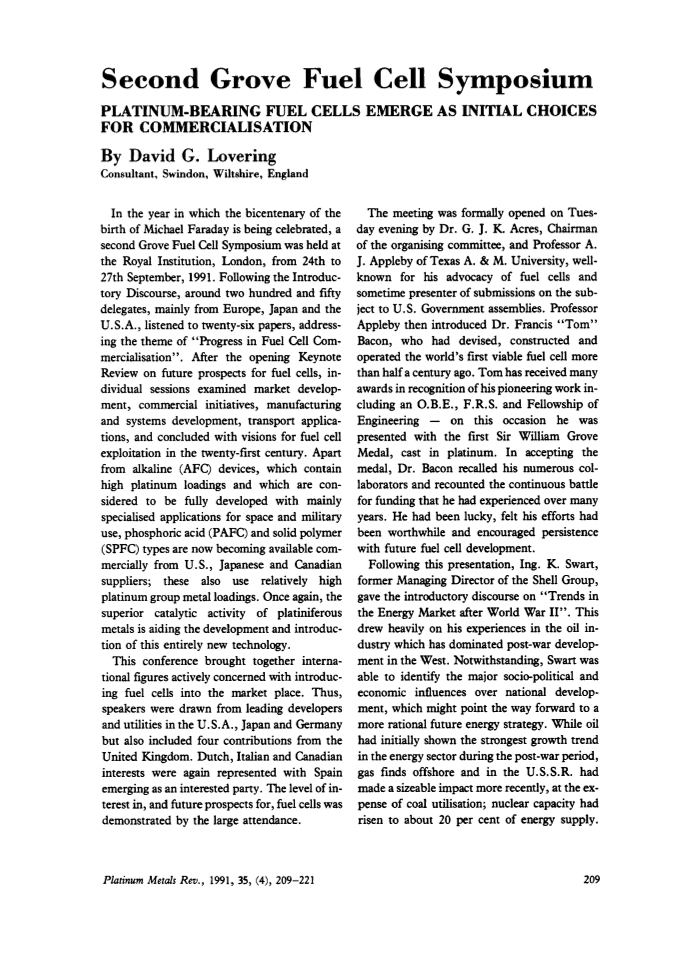



Second Grove Fuel Cell Symposium, Page 1 of 1
< Previous page Next page > /docserver/preview/fulltext/pmr/35/4/pmr0035-0209-1.gif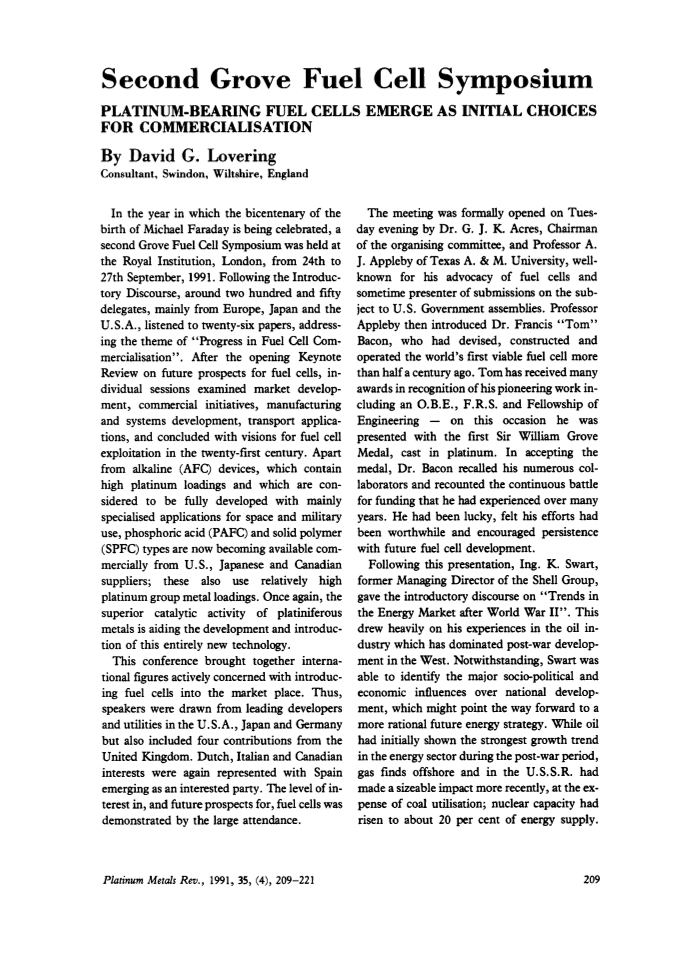
There is no abstract available.
© Johnson Matthey