-
oa Fuel Cells Science and Technology 2010
Scientific advances in fuel cell systems highlighted at the semi-annual event
- Source: Platinum Metals Review, Volume 55, Issue 2, Apr 2011, p. 108 - 116
-
- 01 Jan 2011
Preview this article:
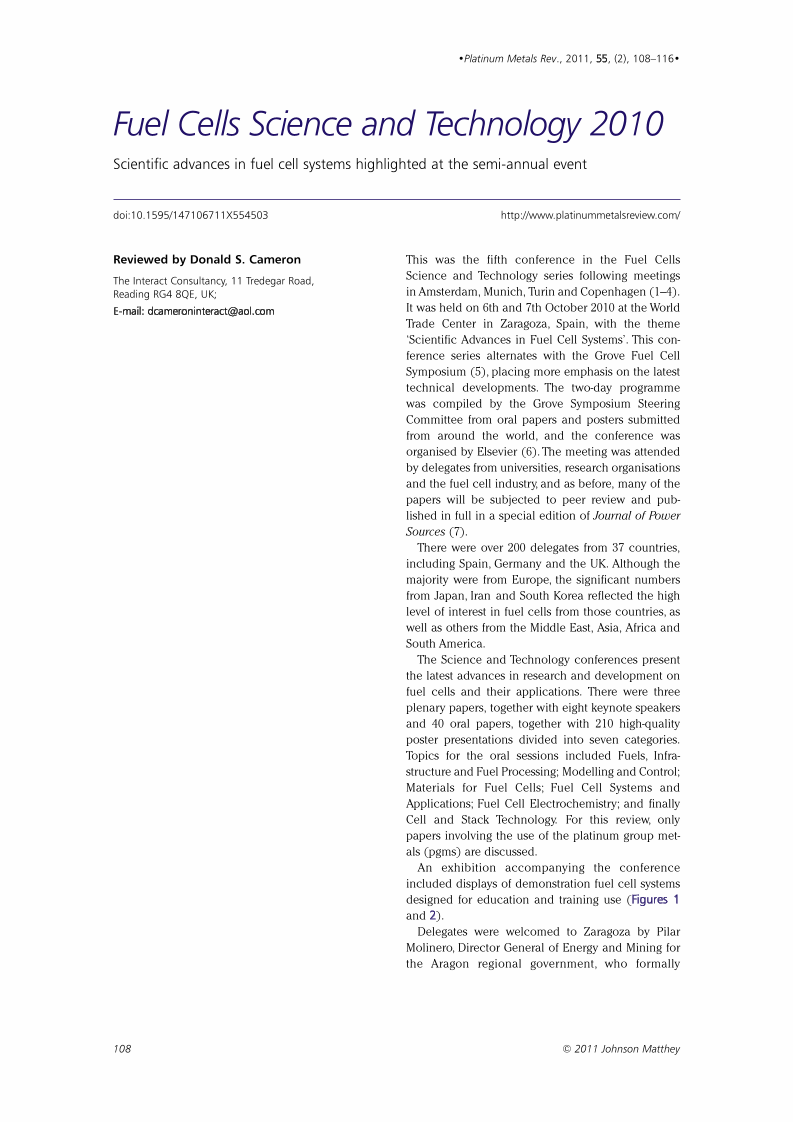



Fuel Cells Science and Technology 2010, Page 1 of 1
< Previous page Next page > /docserver/preview/fulltext/pmr/55/2/PMR-55-2-Cameron-1.gif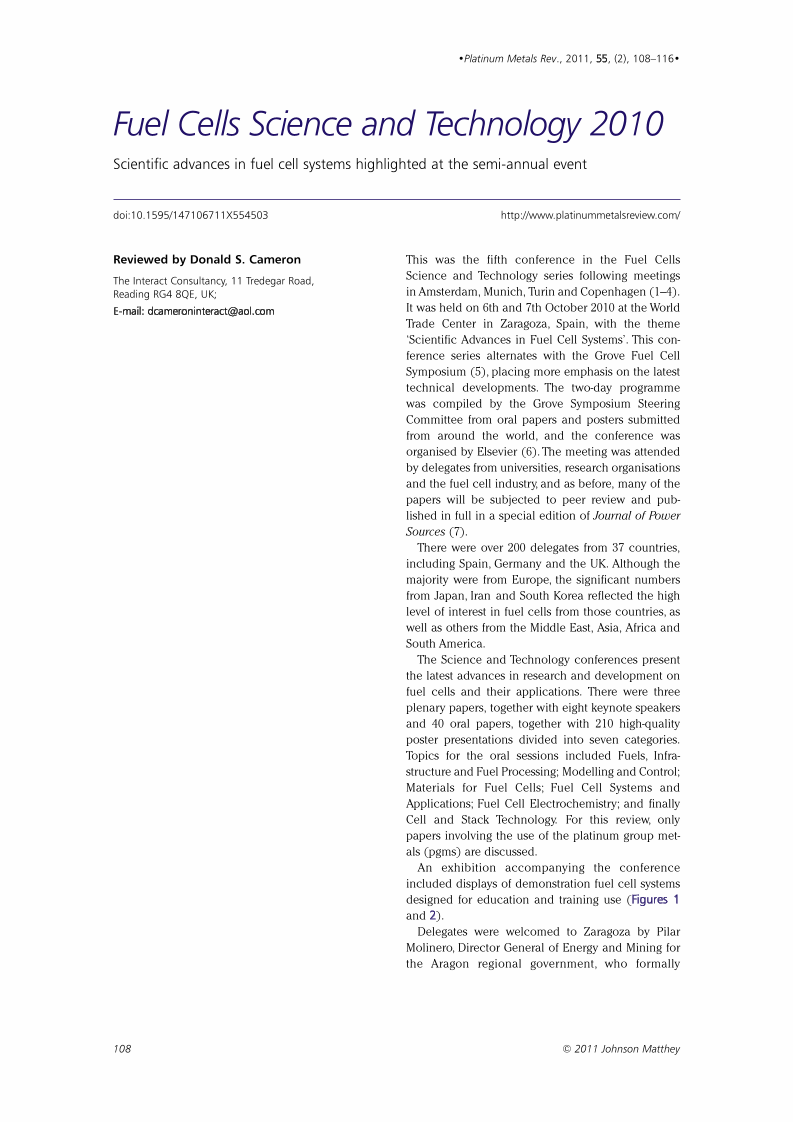
There is no abstract available.
© Johnson Matthey