-
oa “Higher Oxidation State Organopalladium and Platinum Chemistry”
Edited by Allan J. Canty (School of Chemistry, University of Tasmania, Australia), Topics in Organometallic Chemistry, Vol. 35, Springer, Berlin, Heidelberg, Germany, 2011, 186 pages, ISBN: 978-3-642-17428-5, £171.00, €189.95, US$259.00
- Source: Platinum Metals Review, Volume 56, Issue 2, Apr 2012, p. 104 - 109
-
- 01 Jan 2012
Preview this article:
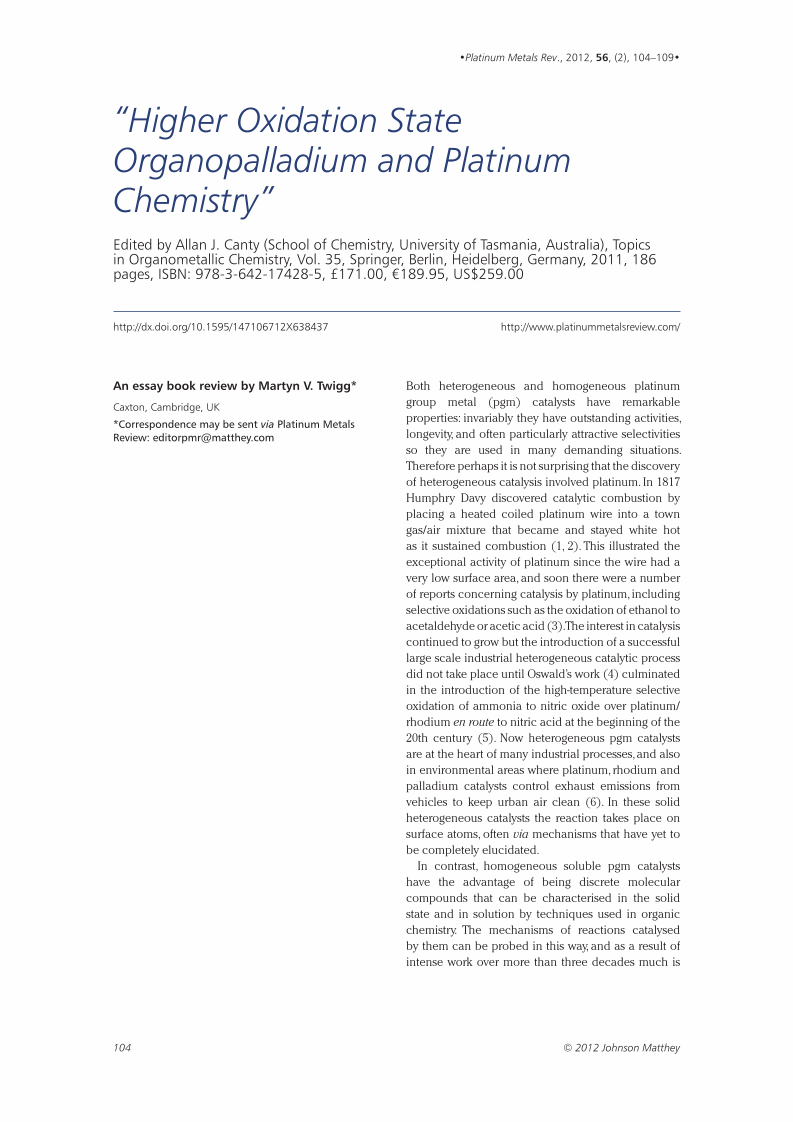



“Higher Oxidation State Organopalladium and Platinum Chemistry”, Page 1 of 1
< Previous page Next page > /docserver/preview/fulltext/pmr/56/2/PMR-56-2-Twigg-1.gif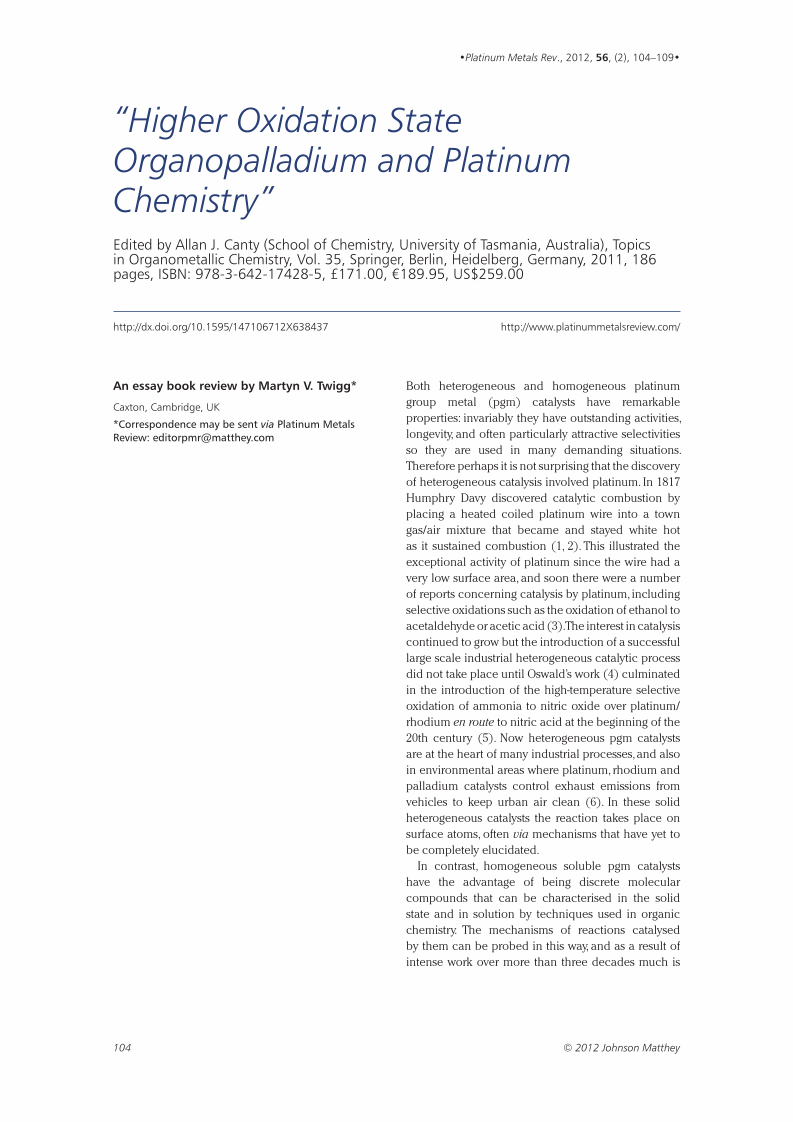
There is no abstract available.
© Johnson Matthey