-
oa Determining Temperature Boundary of the A1→(A1+B2) Phase Transformation in the Copper-55 at% Palladium Alloy Subjected to Severe Plastic Deformation
Higher temperature of phase transformation found for Cu-55 at% Pd alloy
- Source: Johnson Matthey Technology Review, Volume 58, Issue 4, Oct 2014, p. 195 - 201
-
- 01 Jan 2014
Preview this article:
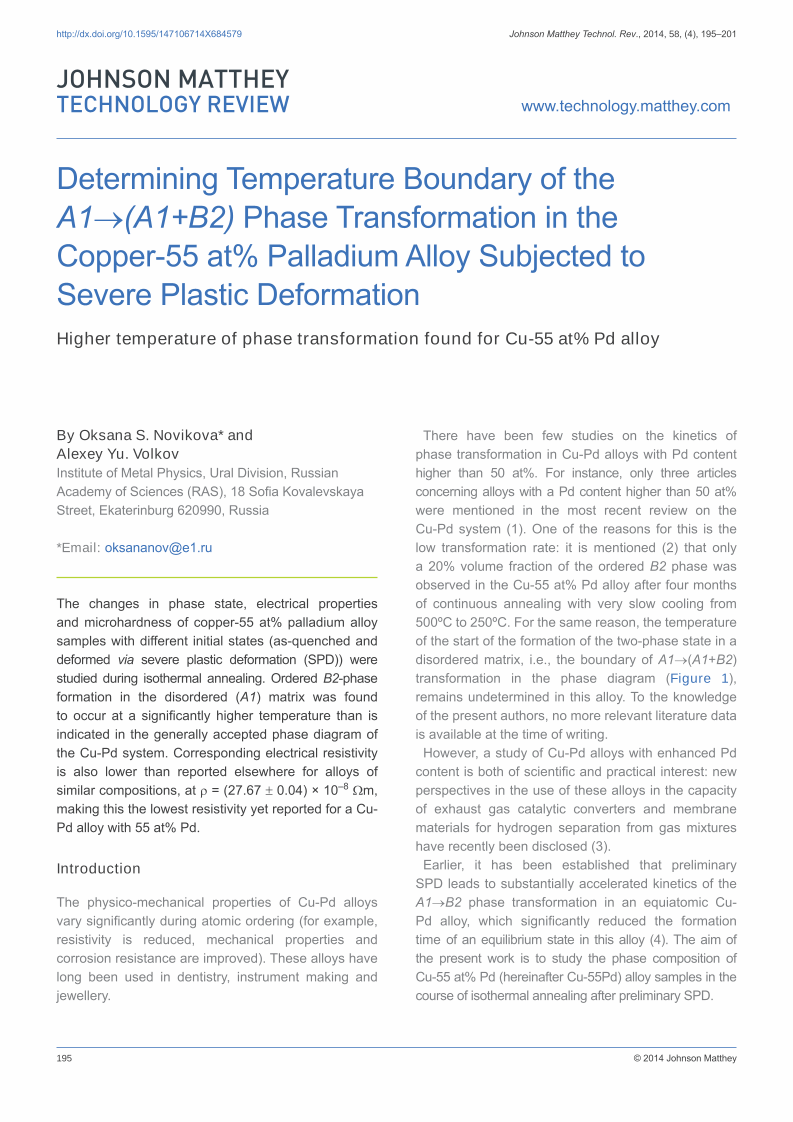



Determining Temperature Boundary of the A1→(A1+B2) Phase Transformation in the Copper-55 at% Palladium Alloy Subjected to Severe Plastic Deformation, Page 1 of 1
< Previous page Next page > /docserver/preview/fulltext/jmtr/58/4/JMTR-58-4-Novikova-1.gif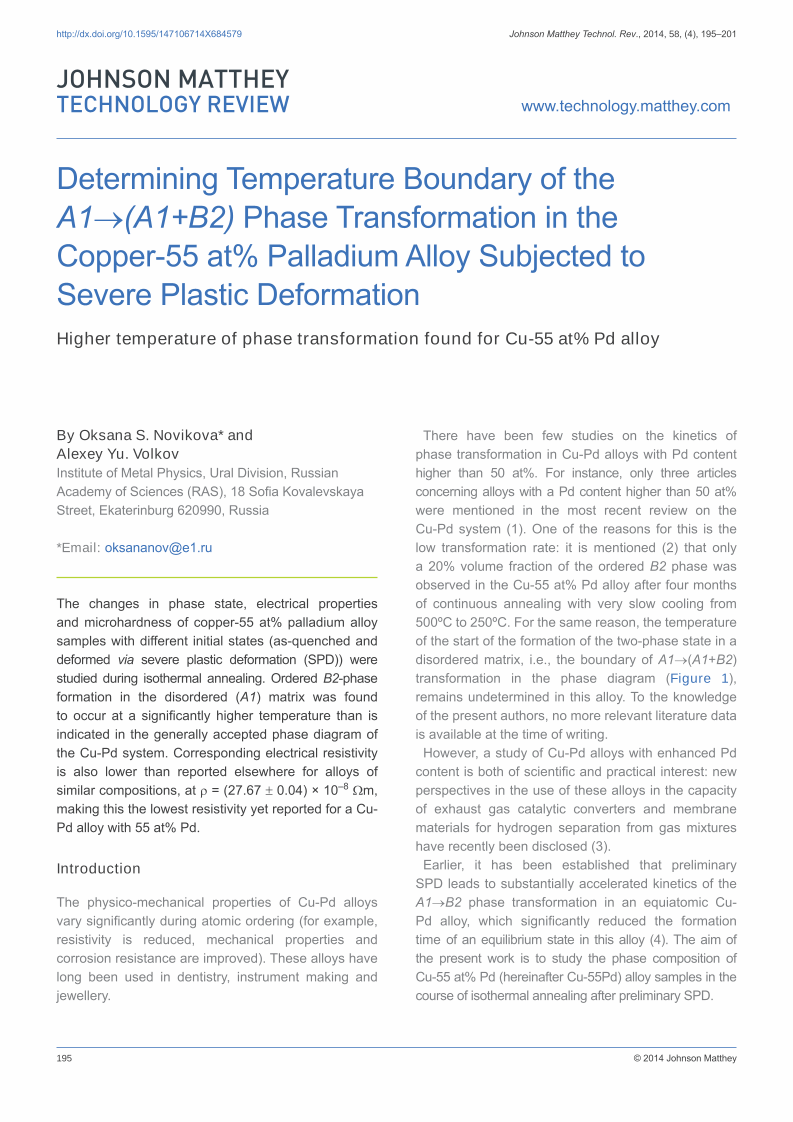
There is no abstract available.
© Johnson Matthey