-
oa In the Lab: Towards a Molecular Level Understanding of Electrochemical Interfaces and Electrocatalytic Reactions
Johnson Matthey Technology Review features new laboratory research
- Source: Johnson Matthey Technology Review, Volume 58, Issue 4, Oct 2014, p. 202 - 204
-
- 01 Jan 2014
Preview this article:
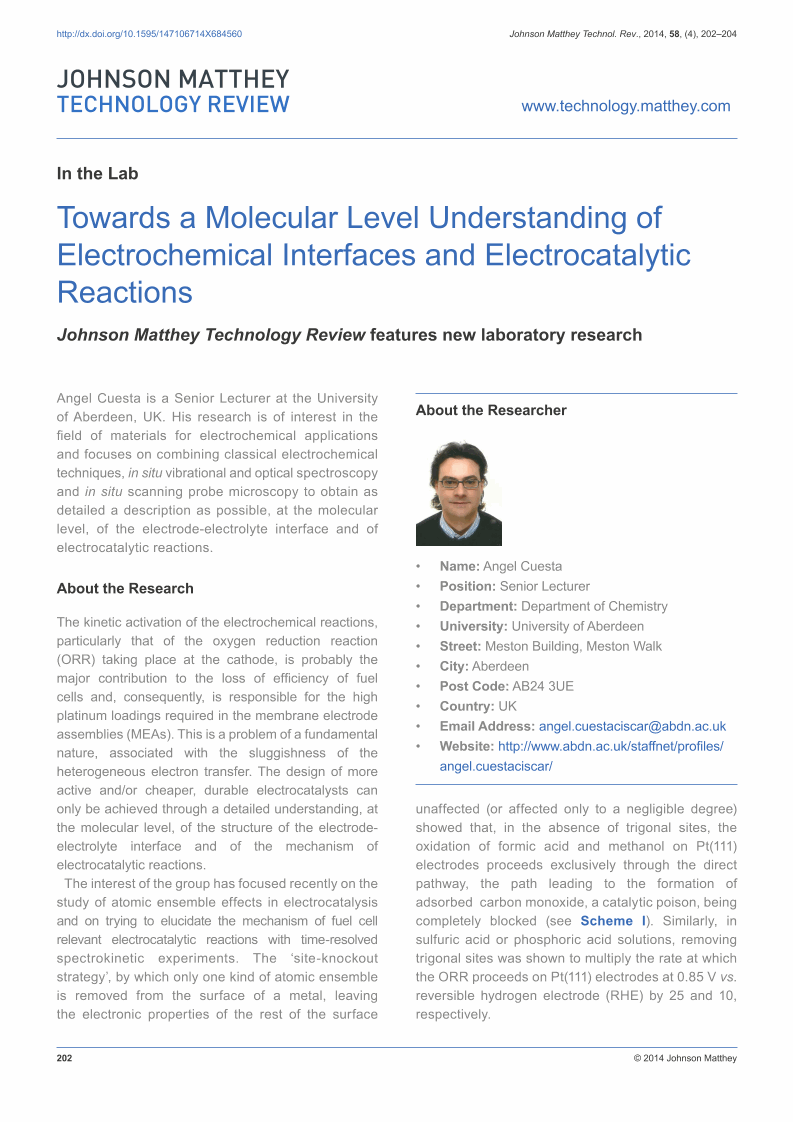



In the Lab: Towards a Molecular Level Understanding of Electrochemical Interfaces and Electrocatalytic Reactions, Page 1 of 1
< Previous page Next page > /docserver/preview/fulltext/jmtr/58/4/JMTR-58-4-In-the-Lab-1.gif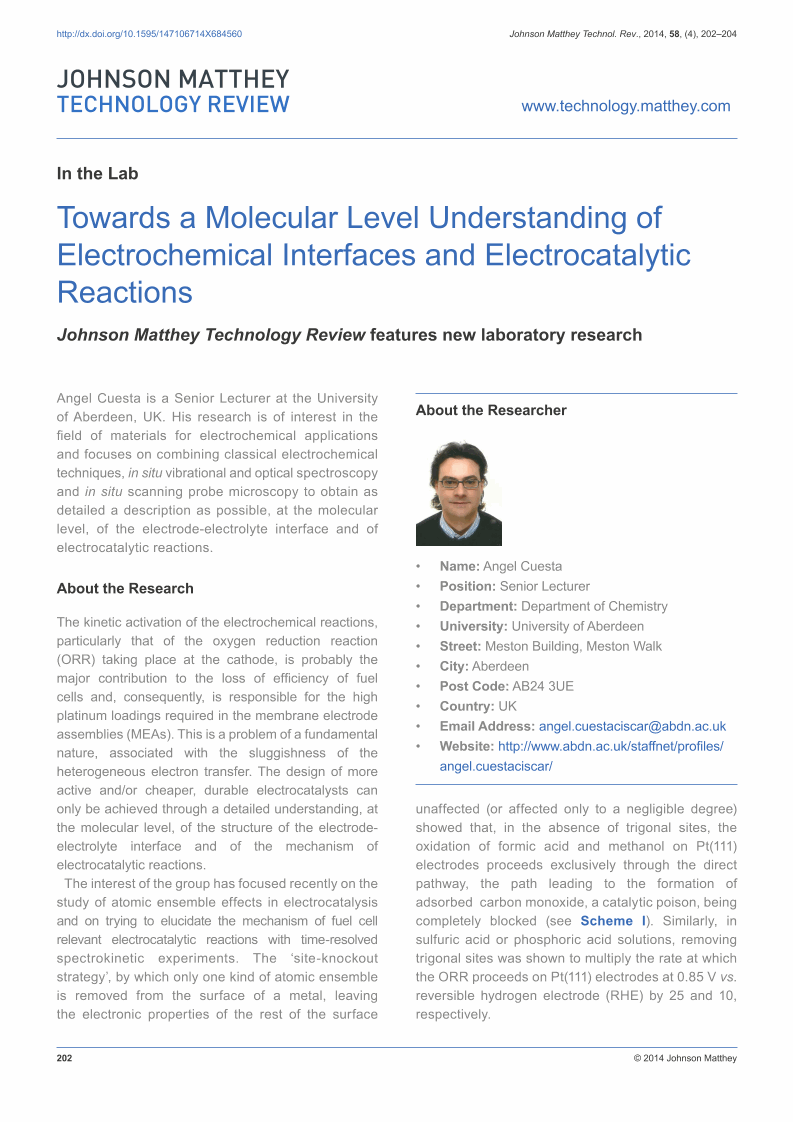
There is no abstract available.
© Johnson Matthey