-
oa “Nanomaterials for Lithium-Ion Batteries: Fundamentals and Applications”
Edited by Rachid Yazami (Nanyang Technological University, Singapore), Pan Stanford Publishing Pte Ltd, USA, 2014, 448 pages, ISBN: 978-981-4316-40-8, £95.00, US$149.95
- Source: Johnson Matthey Technology Review, Volume 59, Issue 1, Jan 2015, p. 26 - 29
-
- 01 Jan 2015
Preview this article:
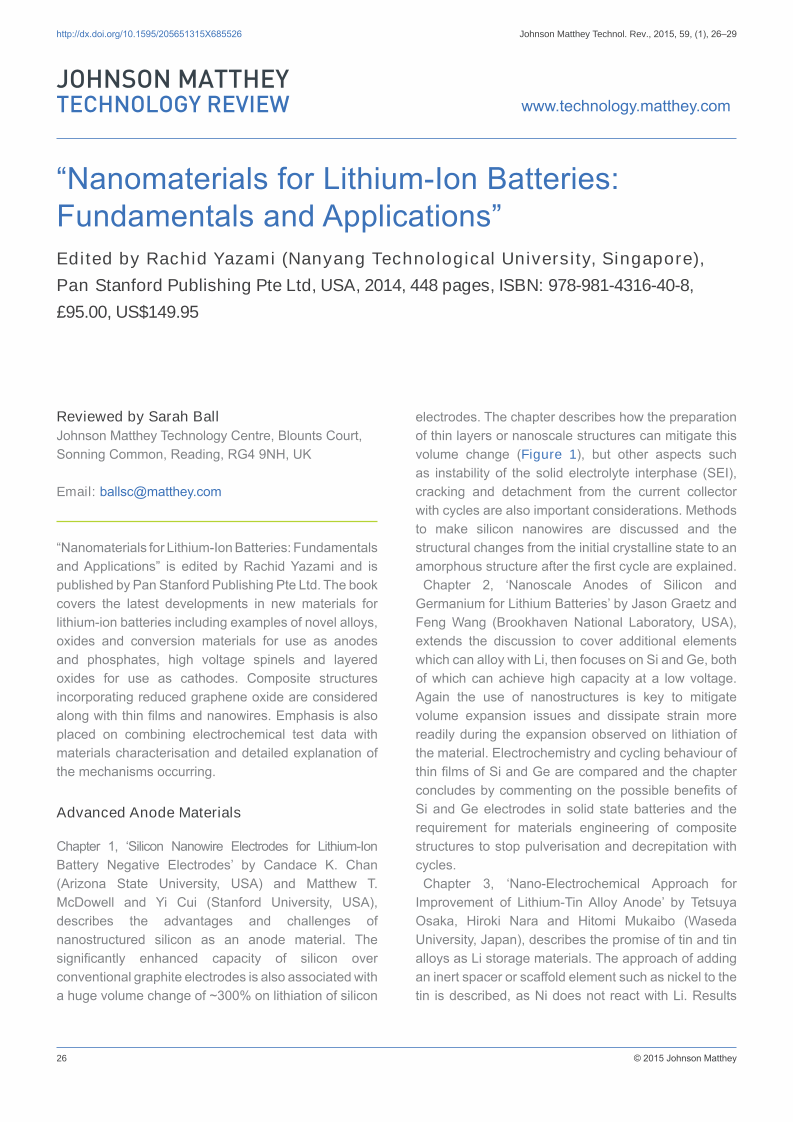



“Nanomaterials for Lithium-Ion Batteries: Fundamentals and Applications”, Page 1 of 1
< Previous page Next page > /docserver/preview/fulltext/jmtr/59/1/JMTR-59-1-Ball-1.gif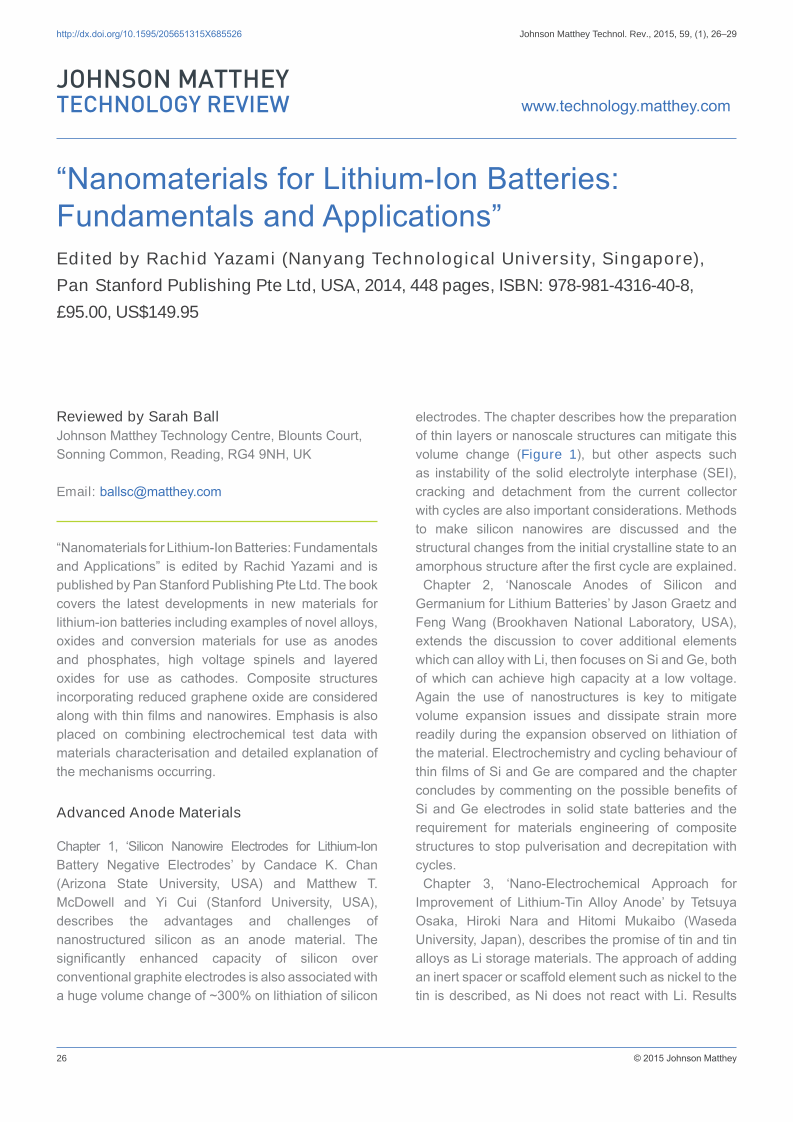
There is no abstract available.
© Johnson Matthey