-
oa “Electrolytes for Lithium and Lithium-Ion Batteries”
Edited by T. Richard Jow, Kang Xu, Oleg Borodin (US Army Research Laboratory, USA) and Makoto Ue (Mitsubishi Chemical Corporation, Japan), Series: Modern Aspects of Electrochemistry, Vol. 58, Springer Science+Business Media, New York, USA, 2014, 476 pages, ISBN: 978-1-4939-0301-6, £117.00, US$179.00, €135.19
- Source: Johnson Matthey Technology Review, Volume 59, Issue 1, Jan 2015, p. 30 - 33
-
- 01 Jan 2015
Preview this article:
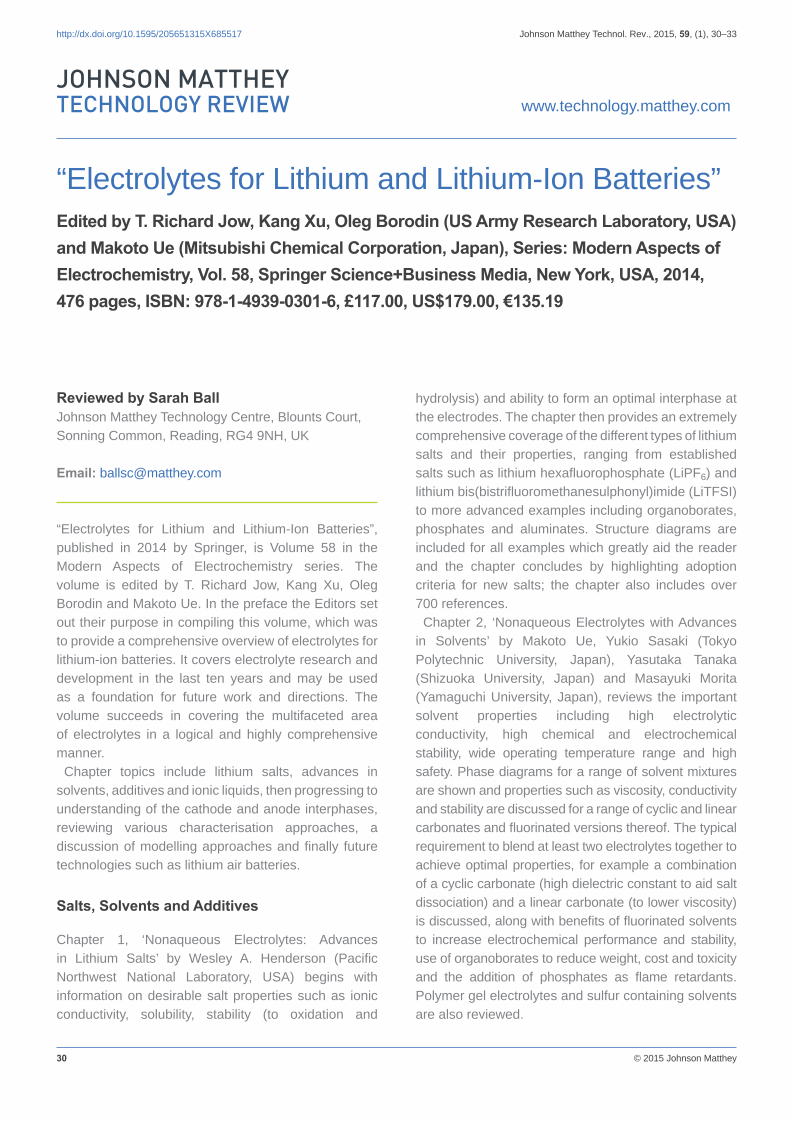



“Electrolytes for Lithium and Lithium-Ion Batteries”, Page 1 of 1
< Previous page Next page > /docserver/preview/fulltext/jmtr/59/1/JMTR-59-1-Ball2-1.gif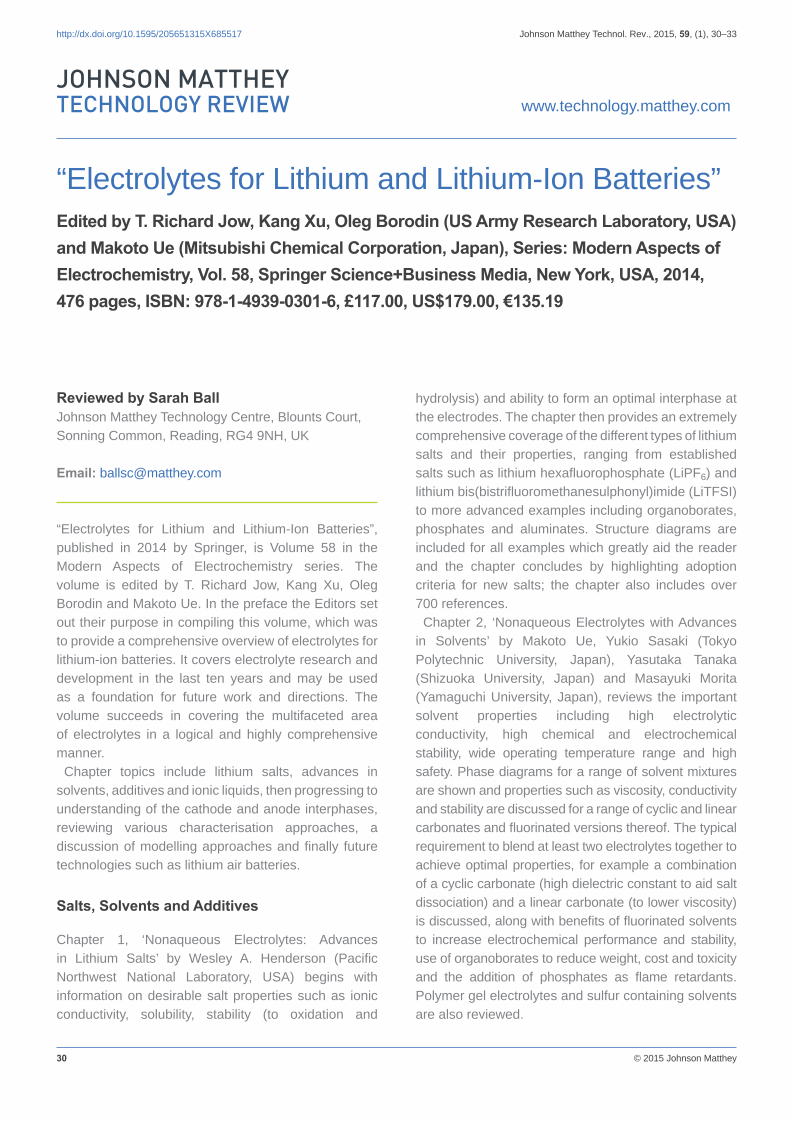
There is no abstract available.
© Johnson Matthey