-
oa Development of Low Temperature Three-Way Catalysts for Future Fuel Efficient Vehicles
Novel alumina/ceria/zirconia mixed oxide with improved thermal stability and oxygen storage capacity enhances low temperature performance of three-way catalysts
- Source: Johnson Matthey Technology Review, Volume 59, Issue 1, Jan 2015, p. 64 - 67
-
- 01 Jan 2015
Preview this article:
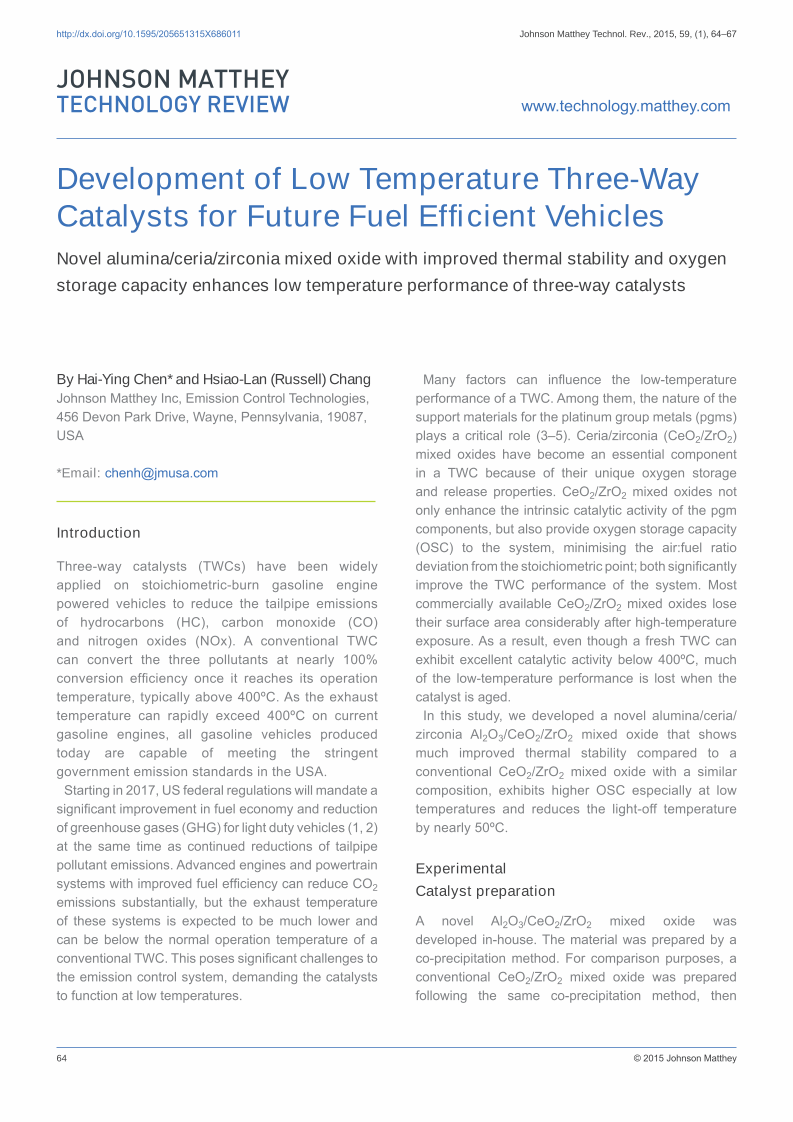



Development of Low Temperature Three-Way Catalysts for Future Fuel Efficient Vehicles, Page 1 of 1
< Previous page Next page > /docserver/preview/fulltext/jmtr/59/1/JMTR-59-1-Chen-1.gif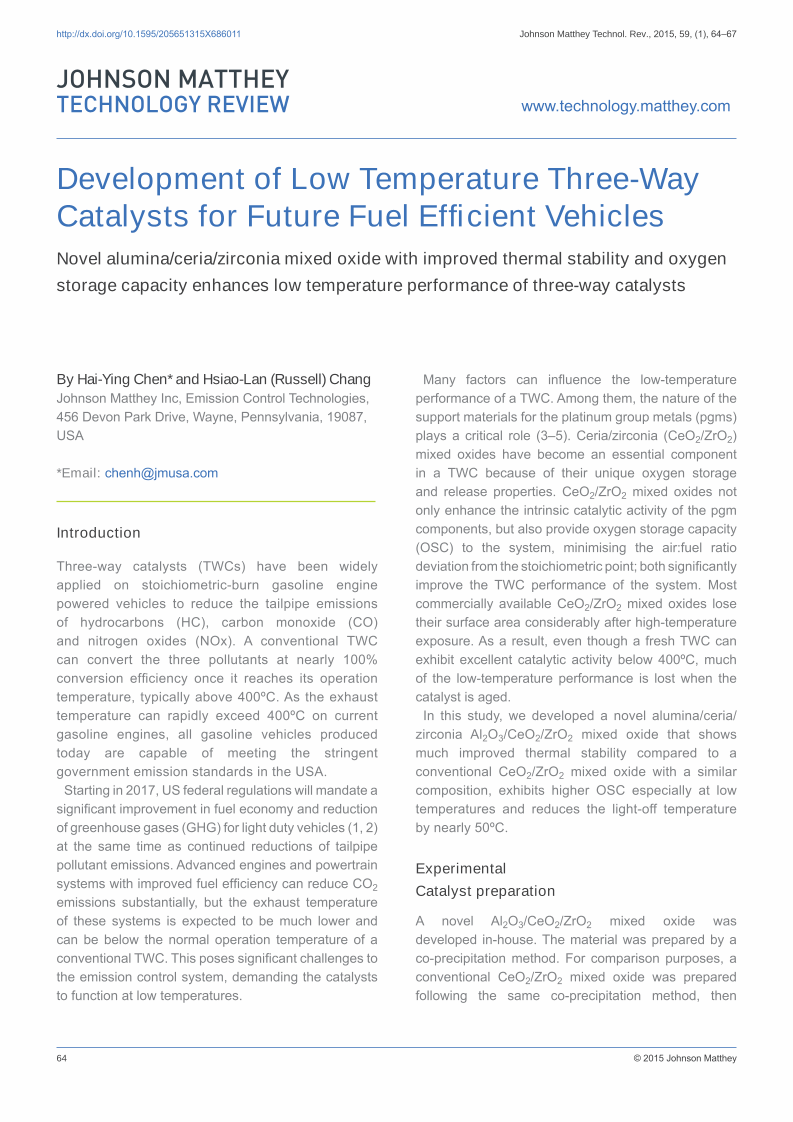
There is no abstract available.
© Johnson Matthey