-
oa “Membrane Technologies for Water Treatment: Removal of Toxic Trace Elements with Emphasis on Arsenic, Fluoride and Uranium”
Edited by Alberto Figoli (Institute on Membrane Technology (ITM-CNR), Rende, Italy), Jan Hoinkis (Karlsruhe University of Applied Sciences, Germany) and Jochen Bundschuh (University of Southern Queensland, Toowoomba, Australia), Taylor & Francis Group, London, UK, 2016, 286 pages, ISBN 978-1-138-02720-6, £127.00, US$159.96
- Source: Johnson Matthey Technology Review, Volume 60, Issue 4, Oct 2016, p. 273 - 276
-
- 01 Jan 2016
Preview this article:
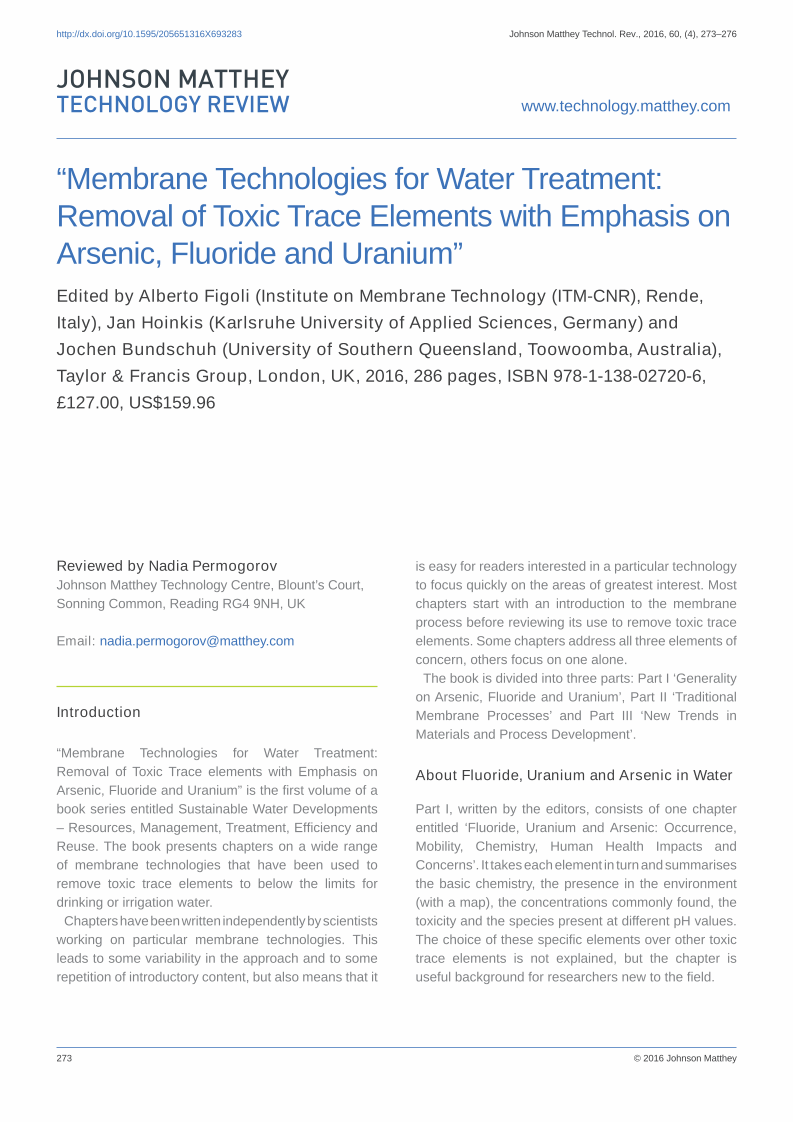



“Membrane Technologies for Water Treatment: Removal of Toxic Trace Elements with Emphasis on Arsenic, Fluoride and Uranium”, Page 1 of 1
< Previous page Next page > /docserver/preview/fulltext/jmtr/60/4/JMTR-60-4-Permogorov-1.gif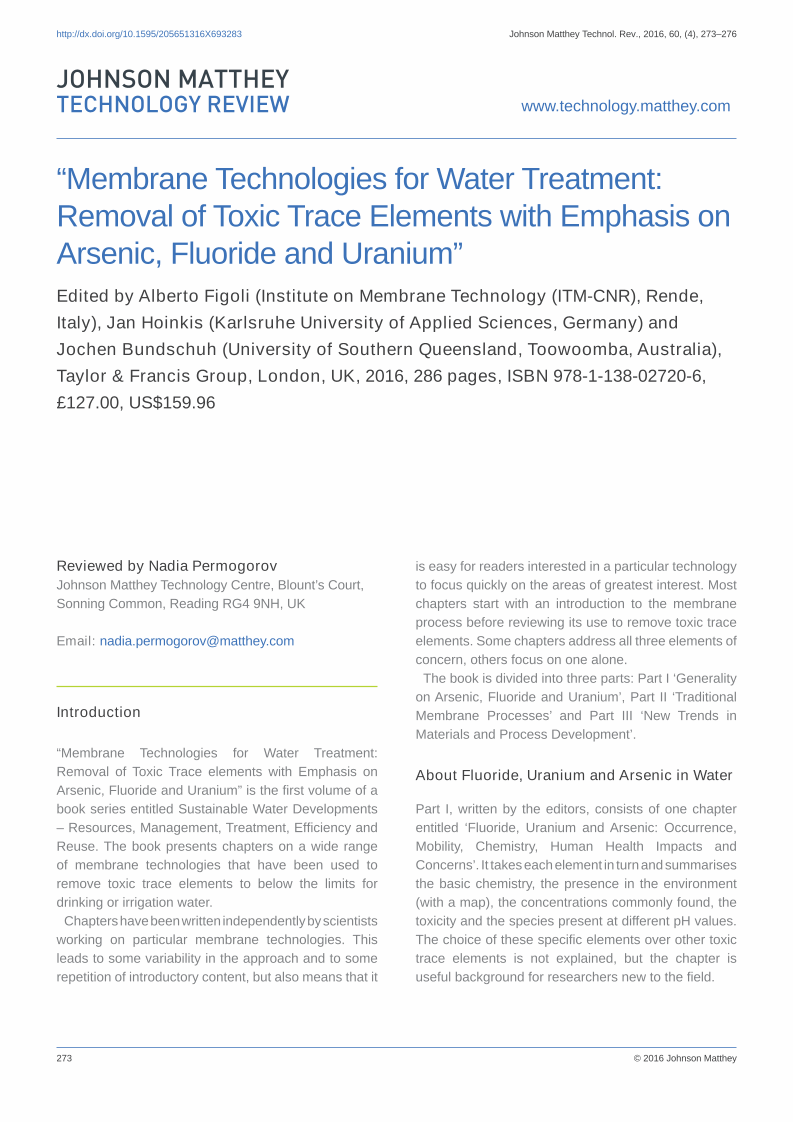
There is no abstract available.
© Johnson Matthey