-
oa “Surgical Tools and Medical Devices” 2nd Edition
Edited by Waqar Ahmed (University of Central Lancashire, UK) and Mark J. Jackson (Kansas State University, USA), Springer International Publishing, Switzerland, 2016, 691 pages, ISBN: 978-3-319-33487-5, £163.00, €227.76, US$249.00
- Source: Johnson Matthey Technology Review, Volume 61, Issue 1, Jan 2017, p. 43 - 46
-
- 01 Jan 2017
Preview this article:
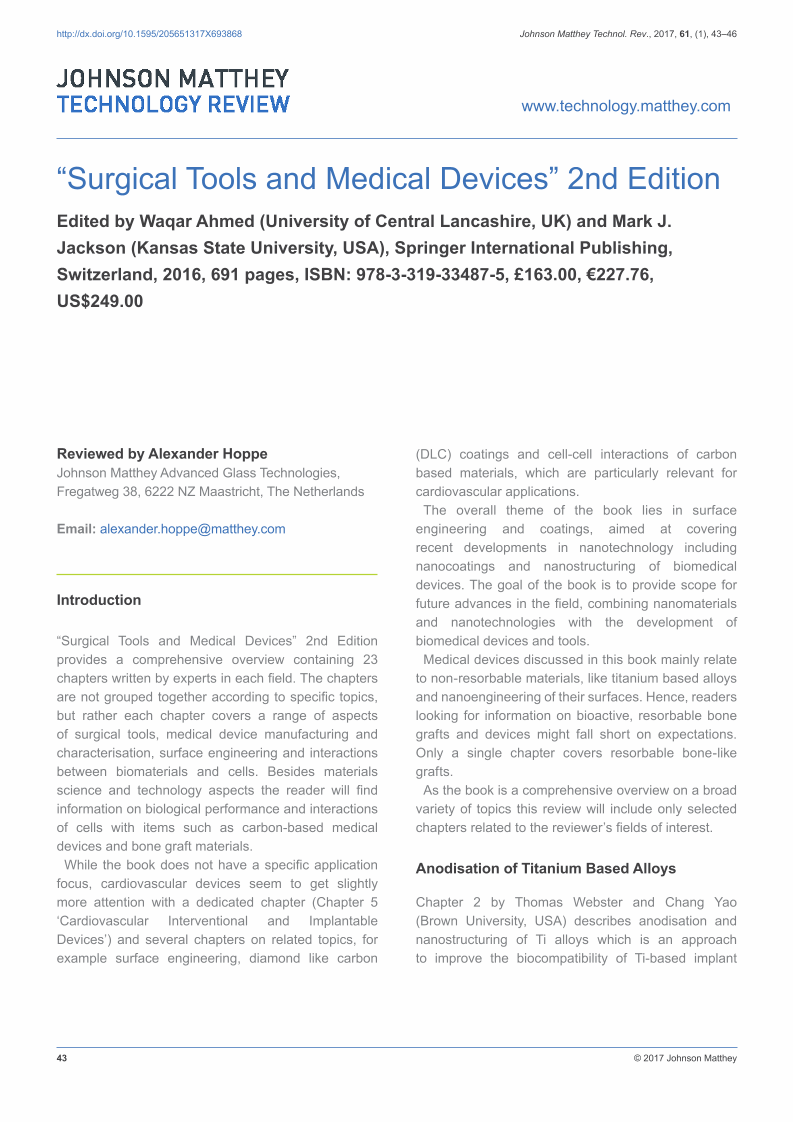



“Surgical Tools and Medical Devices” 2nd Edition, Page 1 of 1
< Previous page Next page > /docserver/preview/fulltext/jmtr/61/1/JMTR-61-1-Hoppe-1.gif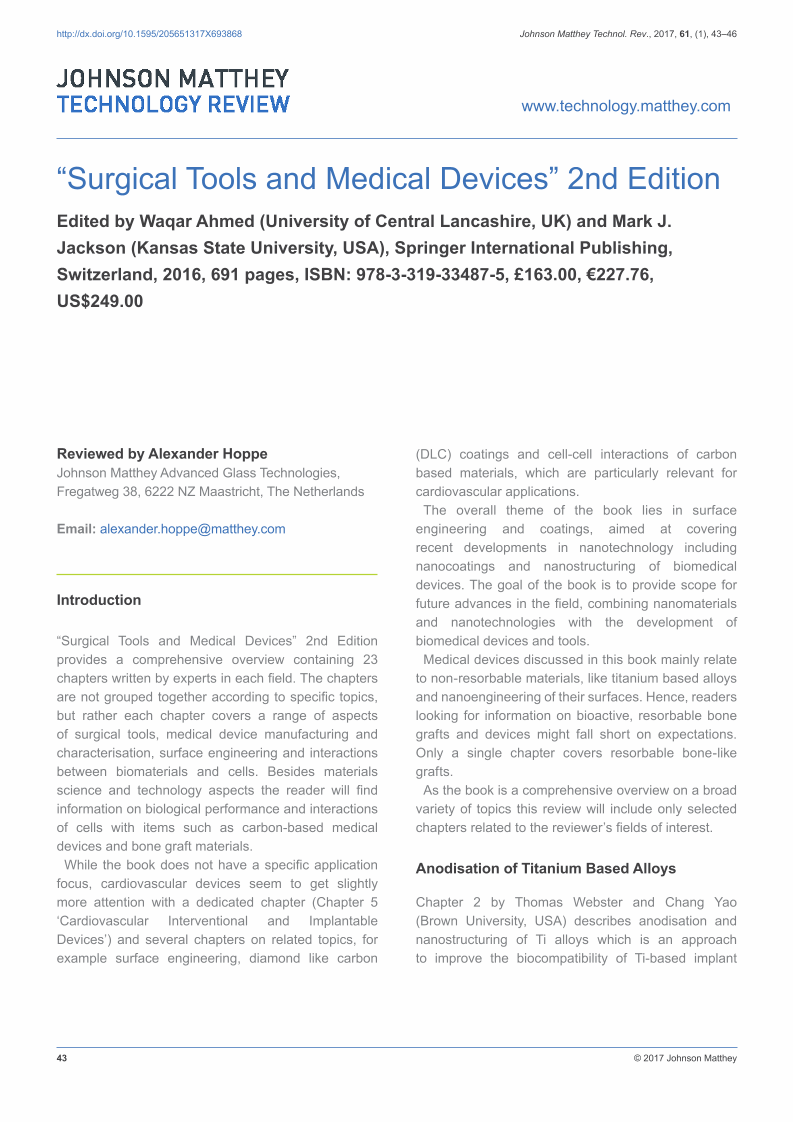
There is no abstract available.
© Johnson Matthey