-
oa Guest Editorial: The Importance of Characterisation Techniques
- Source: Johnson Matthey Technology Review, Volume 60, Issue 2, Apr 2016, p. 88 - 89
-
- 01 Jan 2016
Preview this article:
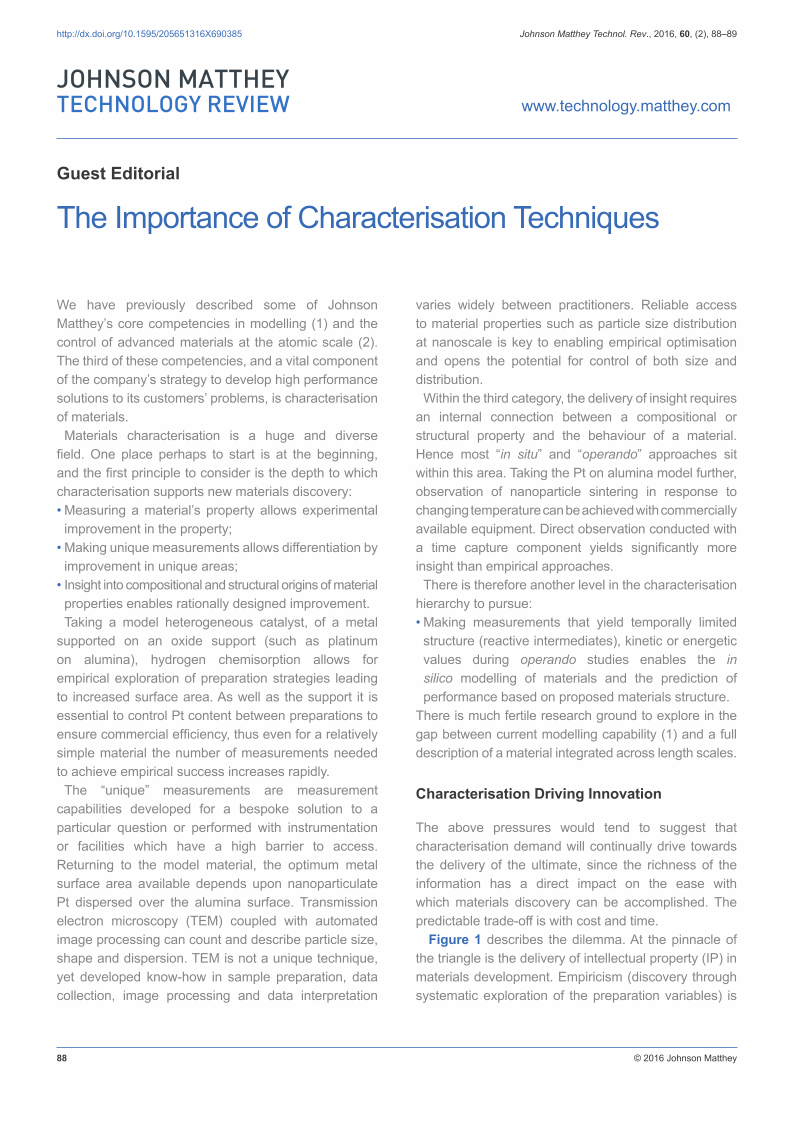



Guest Editorial: The Importance of Characterisation Techniques, Page 1 of 1
< Previous page Next page > /docserver/preview/fulltext/jmtr/60/2/JMTR-60-2-Editorial-1.gif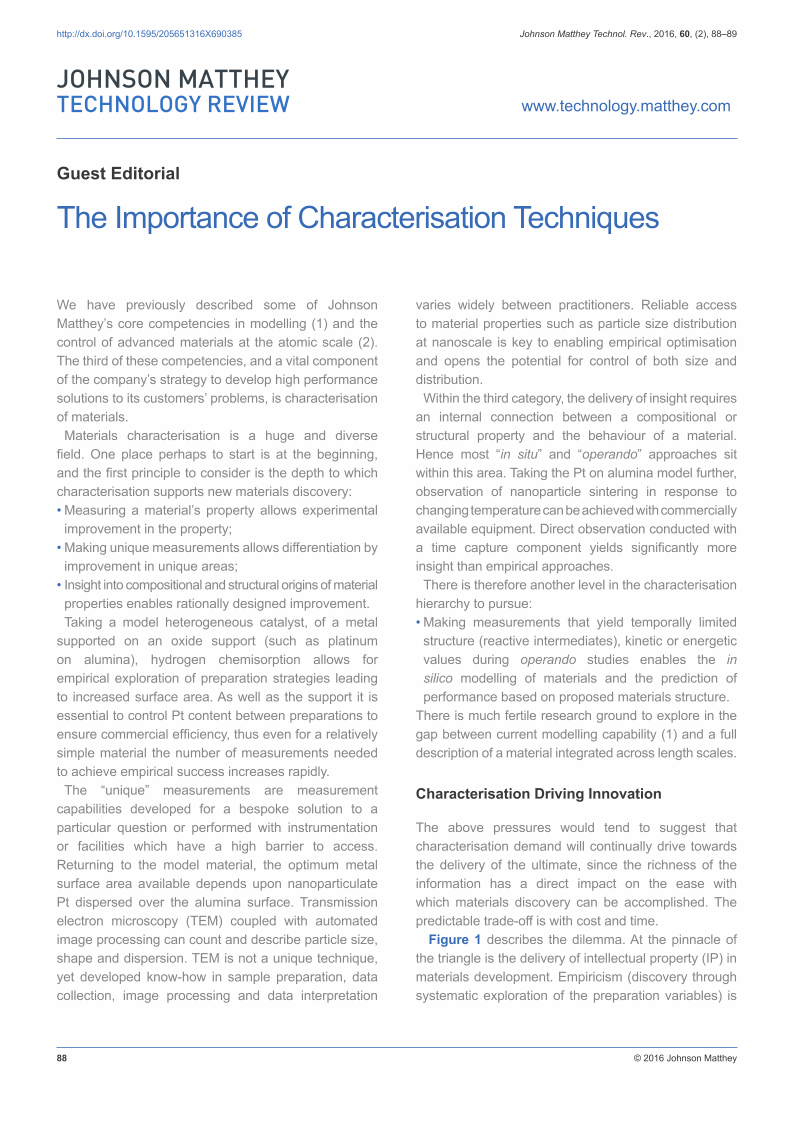
There is no abstract available.
© Johnson Matthey