-
oa Artificial Photosynthesis: Faraday Discussion
Platinum group metals still important for superior photocatalytic activity
- Source: Johnson Matthey Technology Review, Volume 61, Issue 4, Oct 2017, p. 293 - 296
-
- 01 Jan 2017
Preview this article:
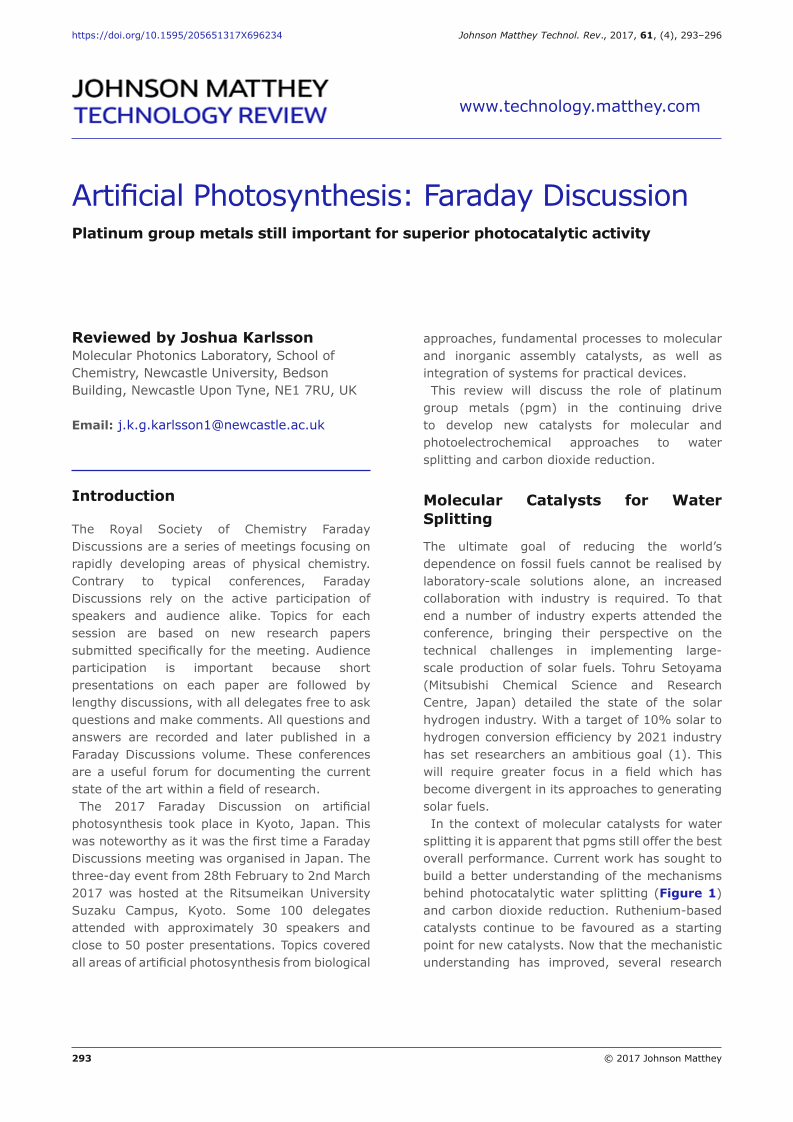



Artificial Photosynthesis: Faraday Discussion, Page 1 of 1
< Previous page Next page > /docserver/preview/fulltext/jmtr/61/4/Karlsson_16a_Imp-1.gif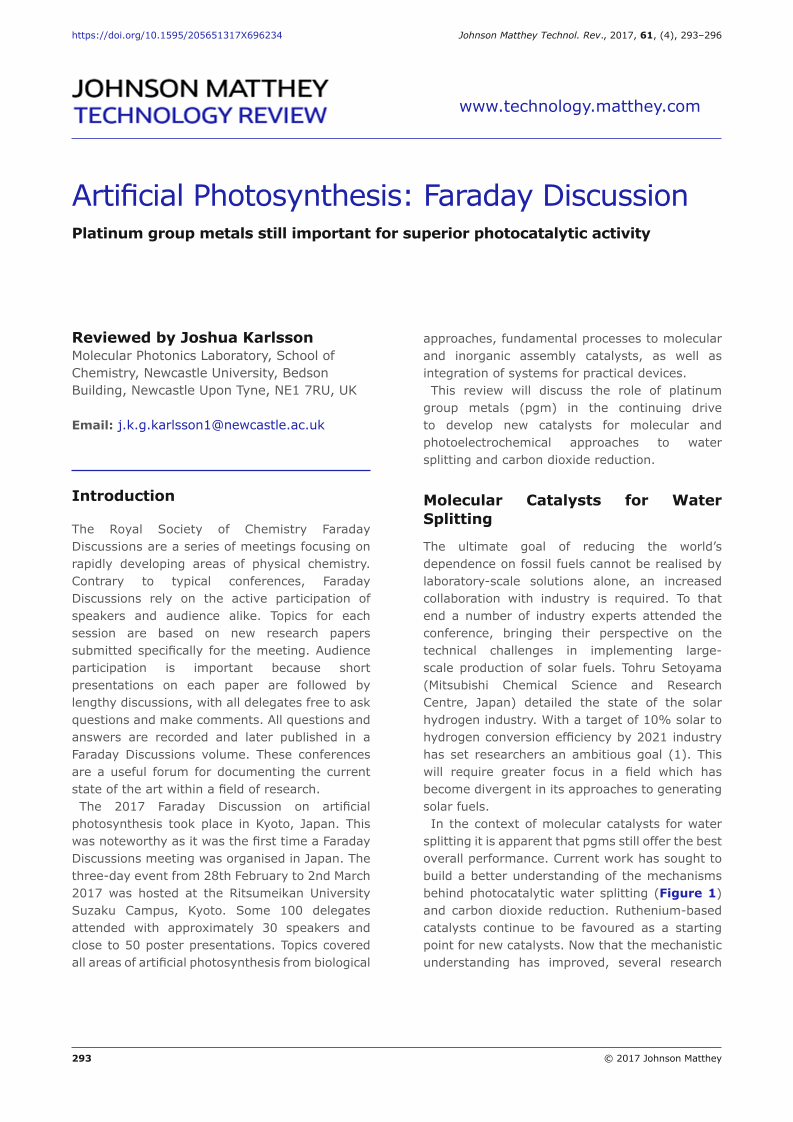
There is no abstract available.
© Johnson Matthey