-
oa “Process Systems Engineering for Pharmaceutical Manufacturing”
Edited by Ravendra Singh (Rutgers, The State University of New Jersey, USA) and Zhinhong Yuan (Tsinghua University, Beijing, China), Computer Aided Chemical Engineering, Volume 41, Elsevier, Amsterdam, The Netherlands, 2018, 674 pages, ISBN: 978-0-444-63963-9, US$187.50, £150.00, €184.57
- Source: Johnson Matthey Technology Review, Volume 63, Issue 4, Oct 2019, p. 292 - 298
-
- 01 Jan 2019
Preview this article:
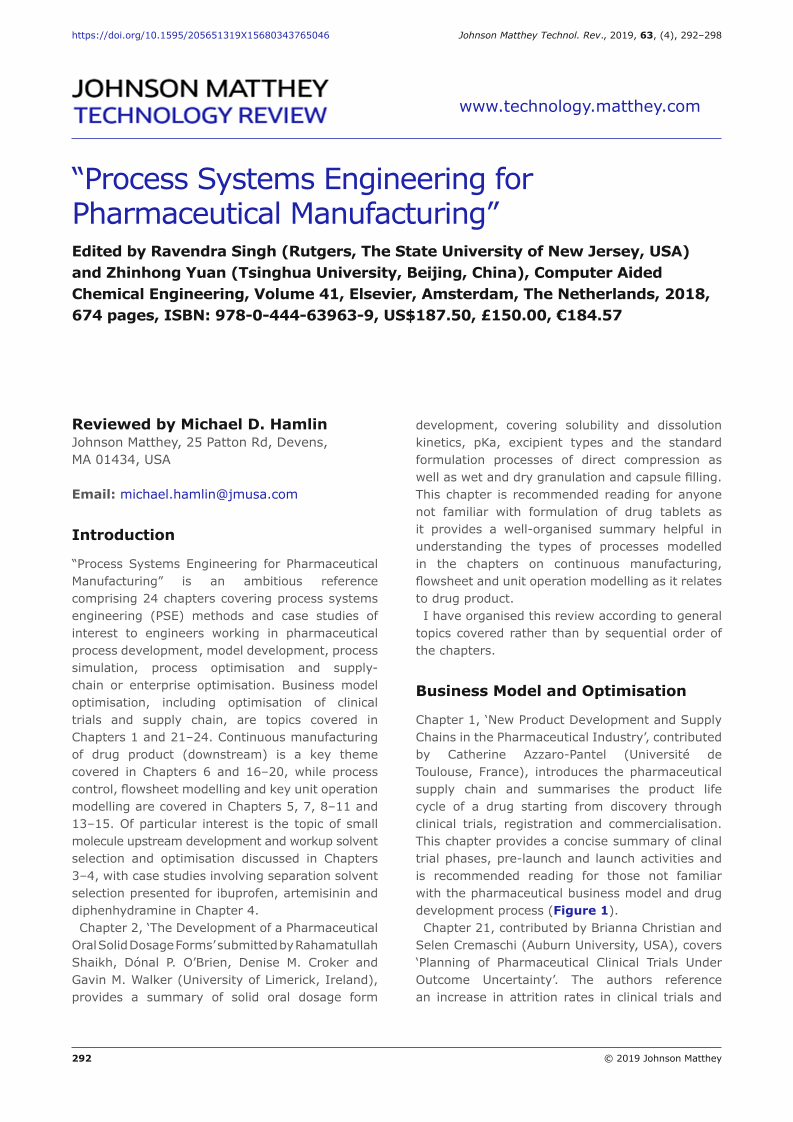



“Process Systems Engineering for Pharmaceutical Manufacturing”, Page 1 of 1
< Previous page Next page > /docserver/preview/fulltext/jmtr/63/4/Hamlin_16a_Imp-1.gif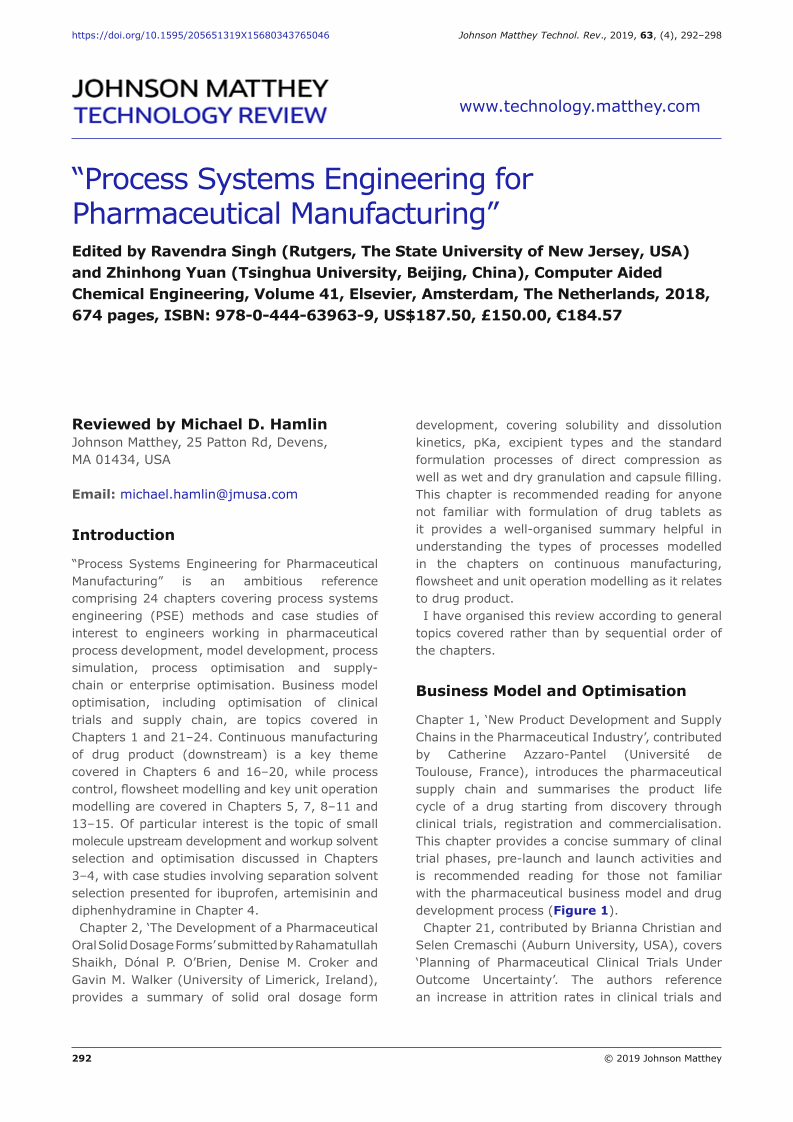
There is no abstract available.
© Johnson Matthey